17.2: Heart Anatomy
- Page ID
- 63478
- Describe the location and position of the heart within the body cavity
- Describe the internal and external anatomy of the heart
- Identify the tissue layers of the heart and pericardium
- Relate the structure of the heart to its function as a double pump
- Compare atrial and ventricular systole and diastole
- Compare systemic circulation to pulmonary circulation
- Trace the pathway of oxygenated and deoxygenated blood through the chambers of the heart
The vital importance of the heart is obvious. If one assumes an average rate of contraction of 75 contractions per minute, a human heart would contract approximately 108,000 times in one day, more than 39 million times in one year, and nearly 3 billion times during a 75-year lifespan. Each of the major pumping chambers of the heart ejects approximately 70 mL of blood per contraction in a resting adult. This would be equal to 5.25 liters of fluid per minute and approximately 14,000 liters per day. Over one year, that would equal 10,000,000 liters or 2.6 million gallons of blood sent through roughly 60,000 miles of vessels.
Location and Position of the Heart
The human heart is located within the thoracic cavity, medially between the lungs in the region known as the mediastinum. The mediastinum also includes the portions of the major blood vessels, the trachea, and the esophagus that are positioned between the lungs medially. Figure \(\PageIndex{1}\) shows the position of the heart within the thoracic cavity. Within the mediastinum, the heart is separated from the other mediastinal structures and is held in place by a tough wrapping known as the pericardium, or pericardial sac. The sac also limits the range of motion of the heart as it beats. The double layered pericardial sac creates a thin space surrounding the heart called the pericardial cavity that is filled with serous fluid to prevent friction as the heart beats.
The dorsal surface of the heart lies near the bodies of the vertebrae and its anterior surface sits deep to the sternum and costal cartilages. The great veins, the superior and inferior venae cavae, and the great arteries, the aorta and pulmonary trunk, are attached to the superior surface of the heart, called the base. The base of the heart is located at the level of the third costal cartilage, as seen in Figure \(\PageIndex{1}\). The inferior tip of the heart, the apex, lies just to the left of the sternum between the junction of the fourth and fifth ribs near their articulation with the costal cartilages. The heart is also rotated slightly around its vertical axis such that the more of the right side of the heart is visible in an anterior view while more of the left side is visible in a posterior view. It is important to remember the position and orientation of the heart when placing a stethoscope on the chest of a patient and listening for heart sounds, and also when looking at images taken from a midsagittal perspective. The slight deviation of the apex to the left is reflected in a depression in the medial surface of the inferior lobe of the left lung, called the cardiac notch.
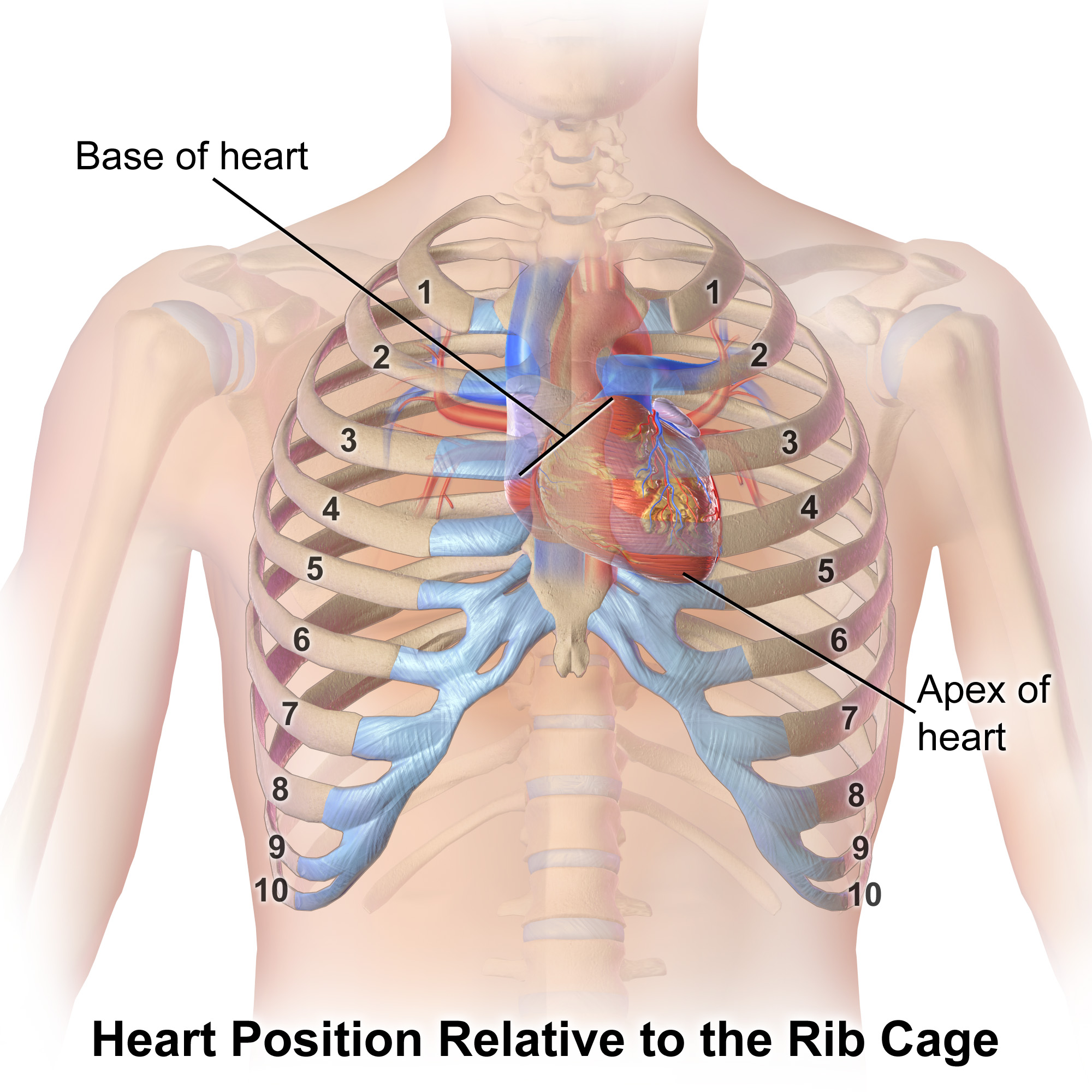
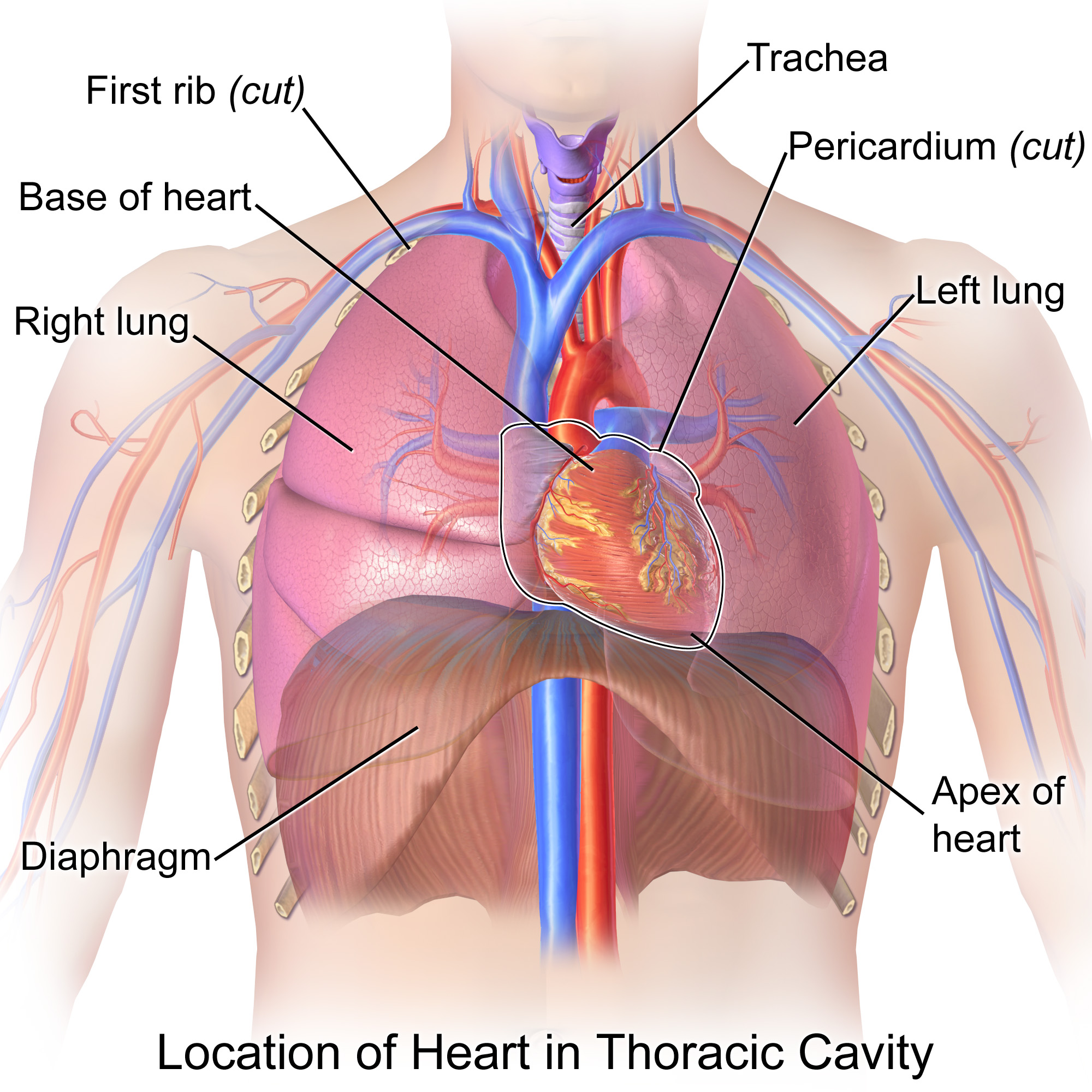
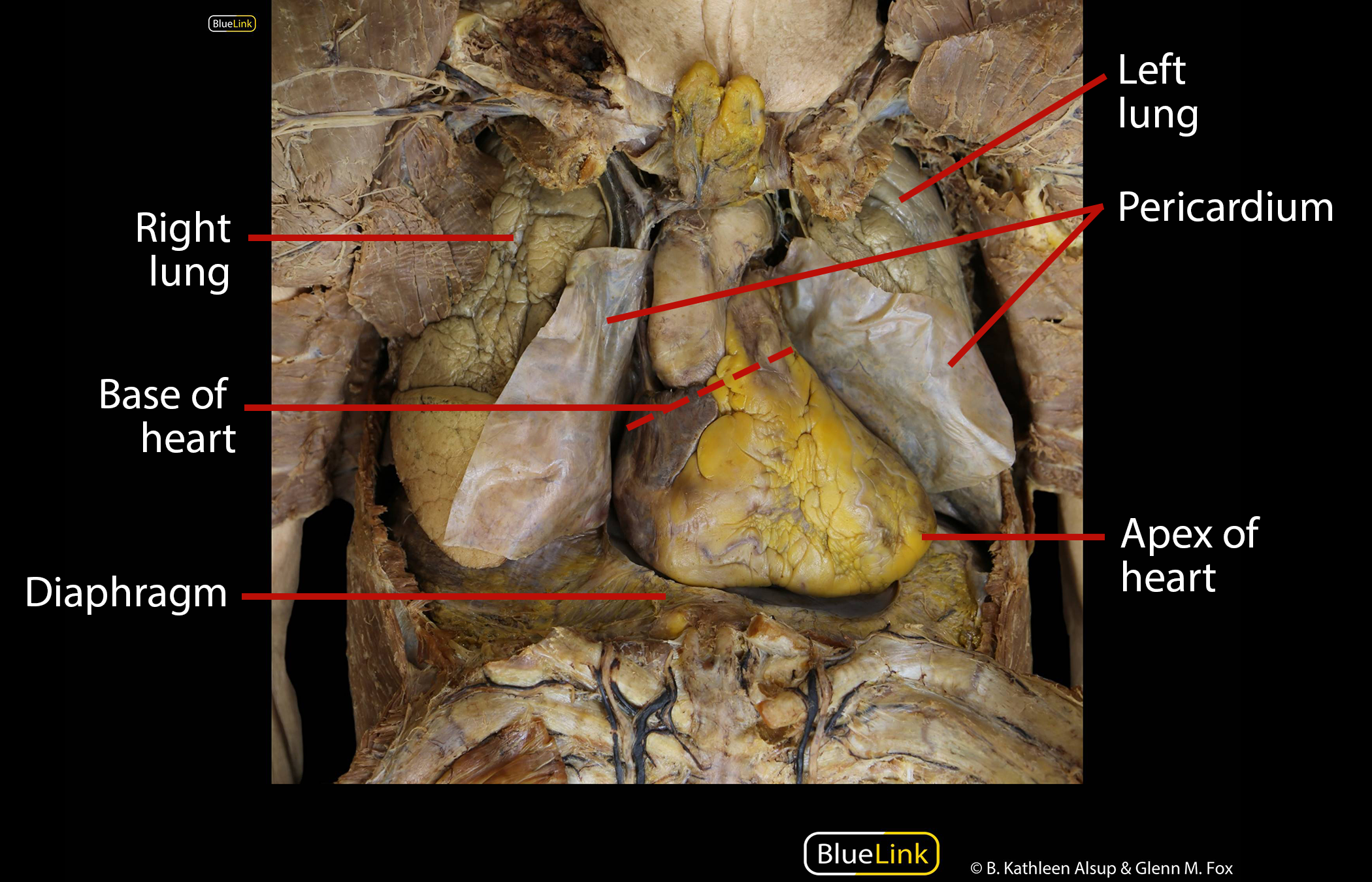
CPR
The position of the heart in the torso between the vertebrae and sternum (see Figure \(\PageIndex{2}\) for the position of the heart within the thorax) allows for individuals to apply an emergency technique known as cardiopulmonary resuscitation (CPR) if the heart of a patient should stop. If one hand is placed over the other in the middle of the chest about two finger-widths superior to the xiphoid process (Figure \(\PageIndex{2}\)), it is possible to manually compress the blood within the heart enough to push some of the blood within it into the pulmonary and systemic circuits. This is particularly critical for the brain, as irreversible damage and death of neurons occur within minutes of loss of blood flow. Current standards call for compression of the chest at least 5 cm deep and at a rate of 100 compressions per minute, a rate equal to the beat in “Staying Alive,” recorded in 1977 by the Bee Gees. If you are unfamiliar with this song, a version is available on www.youtube.com. At this stage, the emphasis is on performing high-quality chest compressions, rather than providing artificial respiration. CPR is generally performed until the patient regains spontaneous contraction or is declared dead by an experienced healthcare professional.
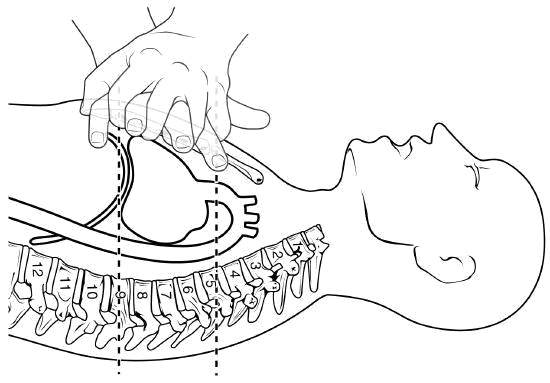
When performed by untrained or overzealous individuals, CPR can result in broken ribs or a broken sternum, and can inflict additional severe damage on the patient. It is also possible, if the hands are placed too low on the sternum, to manually drive the xiphoid process into the liver, a consequence that may prove fatal for the patient. Proper training is essential. This proven life-sustaining technique is so valuable that virtually all medical personnel as well as concerned members of the public should be certified and routinely re-certified in its application. CPR courses are offered at a variety of locations, including colleges, hospitals, the American Red Cross, and some commercial companies. They normally include practice of the compression technique on a mannequin.
The shape of the heart is similar to a pinecone, rather broad at the superior surface (the base, on the posterior side) and tapering to the apex (Figure \(\PageIndex{1}\)). The apex of the heart is inferior and to the left while the base of the heart is superior and posterior.
A typical heart is approximately the size of your fist: 12 cm (5 in) in length, 8 cm (3.5 in) wide, and 6 cm (2.5 in) in thickness. Heart size is dependent on body size, the typical range is 250 to 350 grams (about 1/2 to 3/4 of a pound). The heart of a well-trained athlete, especially one specializing in aerobic sports, can be considerably larger than this. Cardiac muscle responds to exercise in a manner similar to that of skeletal muscle. That is, exercise results in the addition of protein myofilaments that increase the size of the individual cells without increasing their numbers, a concept called hypertrophy. Hearts of athletes can pump blood more effectively at lower rates than those of non-athletes. Enlarged hearts are not always a result of exercise; they can result from pathologies, such as hypertrophic cardiomyopathy. The cause of an abnormally enlarged heart muscle is unknown, but hearts with this condition often have mutation(s) in one of the proteins of the sarcomere, such as myosin or troponin (Popa-Fotea et al, 2019). The condition can be inherited, is often undiagnosed and can cause sudden death in apparently otherwise healthy young people.
Pulmonary and Systemic Circuits
There are two distinct but linked circuits in the human blood circulation called the pulmonary and systemic circuits. Although both circuits transport blood and everything it carries, we can initially view the circuits from the point of view of gases. The pulmonary circuit transports blood to and from the lungs, where it picks up oxygen and delivers carbon dioxide for exhalation. The systemic circuit transports oxygenated blood to virtually all of the tissues of the body and returns relatively deoxygenated blood and carbon dioxide to the heart to be sent back to the pulmonary circulation.
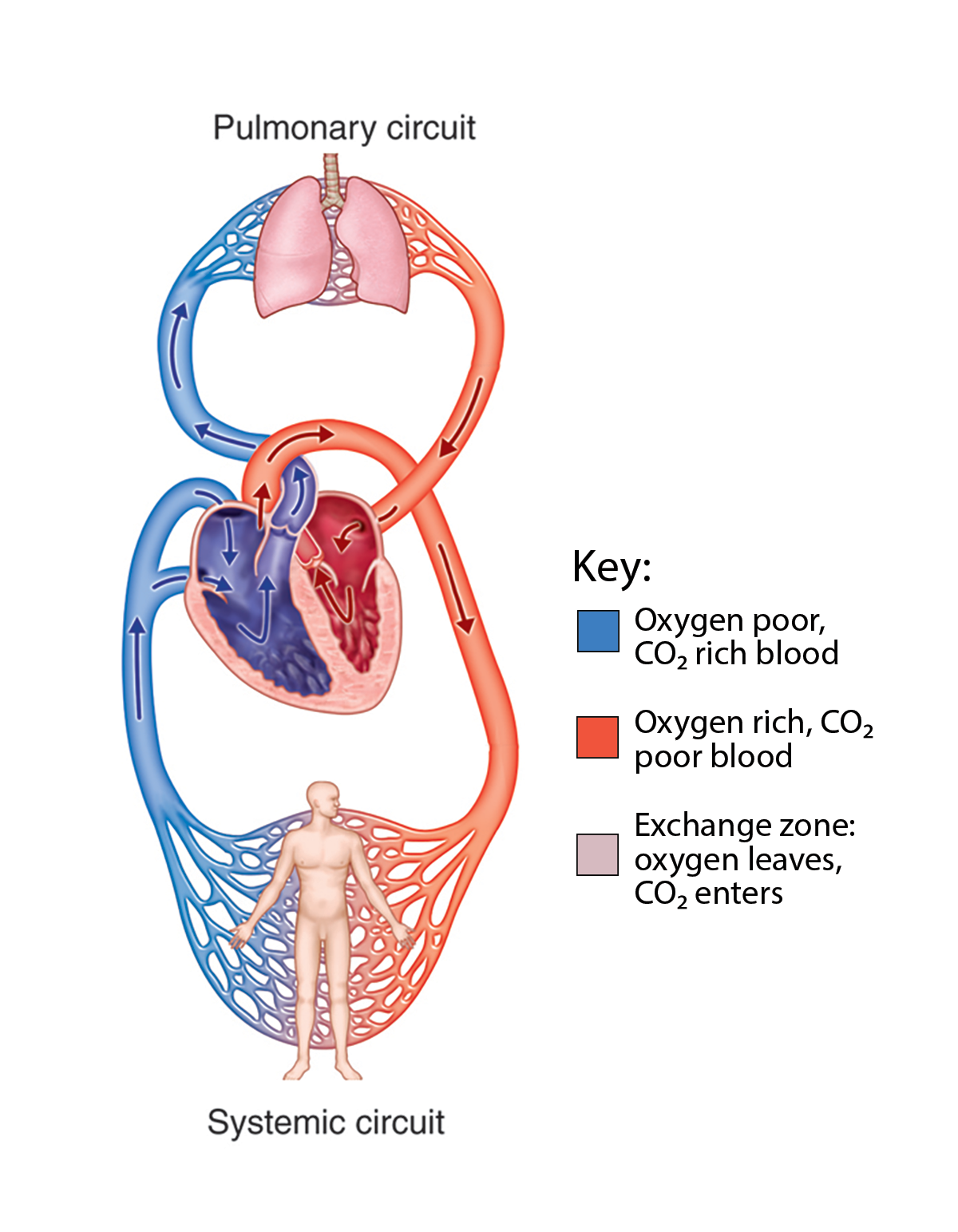
Pericardium and the Heart Wall
The heart is contained within the pericardium. This serous membrane attaches to the large blood vessels superior to the heart and to the diaphragm and helps to anchor the heart in place within the thoracic cavity. The wall of the heart is composed of three layers of unequal thickness. From superficial to deep, these are the epicardium, the myocardium, and the endocardium (see Figure \(\PageIndex{4}\)). The outermost layer of the wall of the heart is also the innermost layer of the pericardium, the epicardium, or the visceral pericardium discussed earlier.
The multi-layered membrane that directly surrounds the heart and defines the pericardial cavity is called the pericardium or pericardial sac. It also surrounds the “roots” of the major vessels, or the areas of closest proximity to the heart. The pericardium, which literally translates as “around the heart,” consists of two distinct sublayers: the sturdy outer fibrous pericardium and the inner serous pericardium. The fibrous pericardium is made of tough, dense irregular connective tissue that protects the heart and maintains its position in the thorax while also limiting the heart's motion during the heartbeat. The more delicate serous pericardium consists of two layers: the parietal pericardium, which is fused to the fibrous pericardium, and an inner visceral pericardium, or epicardium, which is fused to the heart and is part of the heart wall. The pericardial cavity, filled with lubricating serous fluid, lies between the epicardium and the parietal pericardium. The serous layers of the pericardium consist of a simple squamous epithelium anchored by a layer of areolar connective tissue. The areolar connective tissue connects the parietal pericardium to the fibrous pericardium and it connects the epicardium to the myocardium. The epithelial tissue secretes the lubricating serous fluid that fills the pericardial cavity and reduces friction as the heart contracts. Adipose connective tissue can also be found in the epicardium, particularly in the areas where blood vessels run along the heart's surface. Figure \(\PageIndex{4}\) illustrates the pericardial membrane and the layers of the heart.
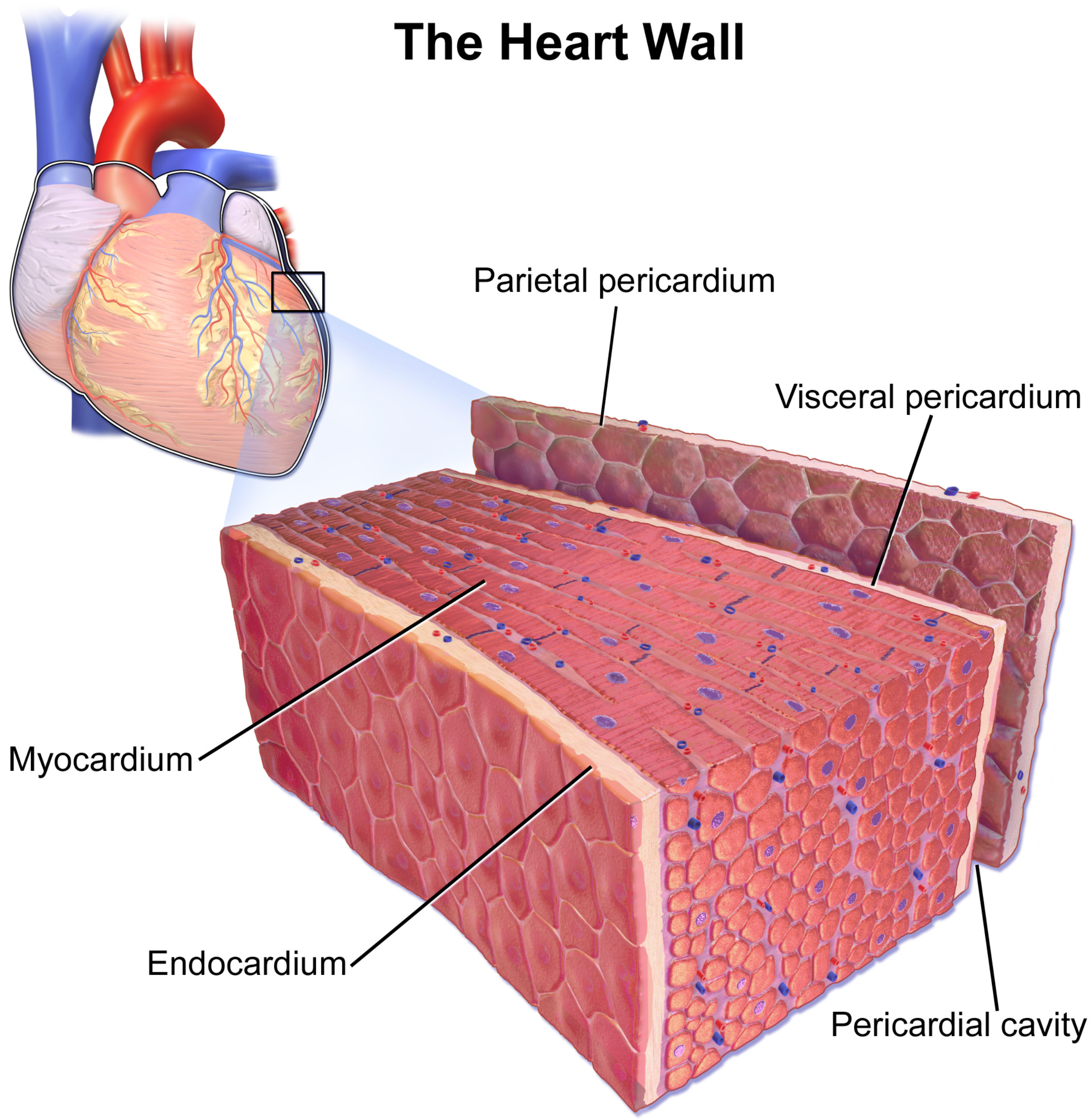
The middle and thickest layer is the myocardium. Composed primarily of cardiac muscle, with small amounts of endomysium, the contractile force of the myocardium propels blood forward through the pulmonary and systemic circuits. As with skeletal muscle, the amount of force generated increases with increasing numbers of myofibers.
The myocardium is built upon a framework of dense connective tissue called the cardiac skeleton (covered in detail later in this section). It is the contraction of the myocardium that pumps blood through the heart and into the major arteries. The muscle pattern is elegant and complex, as the muscle cells swirl and spiral to form the chambers of the heart. To create this complex 3D structure, cardiac muscle cells approximately follow a figure 8 pattern around the atria and around the roots of the great vessels. Deeper ventricular muscles also form a figure 8 around the two ventricles and proceed toward the apex. More superficial layers of ventricular muscle wrap around both ventricles. This complex swirling pattern allows the heart to pump blood more effectively by decreasing the size of each interior chamber during contraction. Figure \(\PageIndex{5}\) illustrates the arrangement of muscle cells.
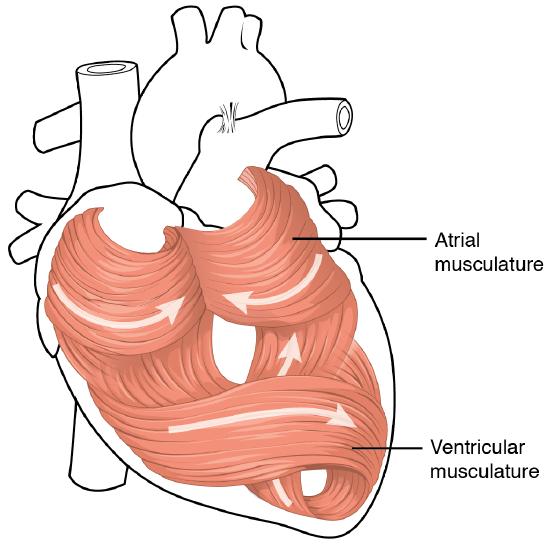
Although the ventricles on the right and left sides pump the same amount of blood per contraction, the muscle of the left ventricle is much thicker and better developed than that of the right ventricle. In order to overcome the high resistance required to pump blood into the long systemic circuit, the left ventricle must generate a great amount of pressure. The right ventricle does not need to generate as much pressure, since the pulmonary circuit is shorter and provides less resistance. Figure \(\PageIndex{6}\) illustrates the differences in muscular thickness needed for each of the ventricles.
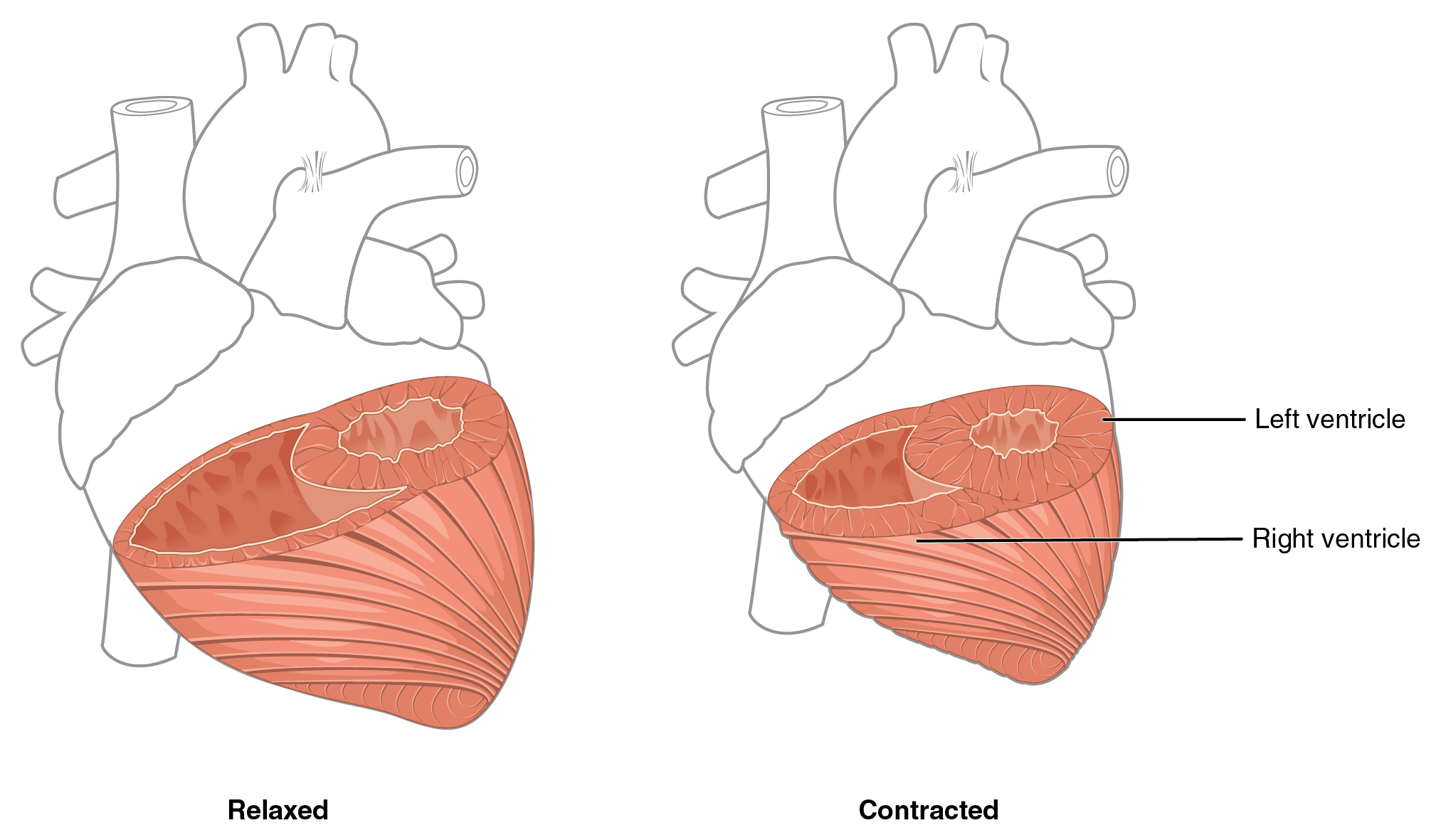
The innermost layer of the heart wall, the endocardium, is joined to the myocardium with a thin layer of areolar connective tissue. The endocardium lines the chambers where the blood circulates and covers the heart valves. It is made of simple squamous epithelium called endothelium, which is continuous with the endothelial lining of the blood vessels (Figure \(\PageIndex{4}\)).
Heart: Cardiac Tamponade
If excess fluid builds within the pericardial space, it can lead to a condition called cardiac tamponade, or pericardial tamponade. With each contraction of the heart, more fluid—in most instances, blood—accumulates within the pericardial cavity. In order to fill with blood for the next contraction, the heart must relax. However, the excess fluid in the pericardial cavity puts pressure on the heart and prevents full relaxation, so the chambers within the heart contain slightly less blood as they begin each heart cycle. Over time, less and less blood is ejected from the heart. If the fluid builds up slowly, as in hypothyroidism, the pericardial cavity may be able to expand gradually to accommodate this extra volume. Some cases of fluid in excess of one liter within the pericardial cavity have been reported. Rapid accumulation of as little as 100 mL of fluid following trauma may trigger cardiac tamponade. Other common causes include myocardial rupture, pericarditis, cancer, or even cardiac surgery. Removal of this excess fluid requires insertion of drainage tubes into the pericardial cavity. Premature removal of these drainage tubes, for example, following cardiac surgery, or clot formation within these tubes are causes of this condition. Untreated, cardiac tamponade can lead to death.
Surface Features of the Heart
The heart is composed of four hollow chambers: two atria and two ventricles. The thin walled right and left atria are the two superior chambers. While both atria are visible from the anterior, the majority of the left atrium is seen on the posterior aspect ((Figure \(\PageIndex{7}\)). Each atrium has a superficial flap-like extension called an auricle because its shape resembles the external ear of a human (Figure \(\PageIndex{8}\)). You may also hear them referred to as atrial appendages. The auricles can increase the atrial capacity at times of exertion by filling with blood and, thus, relieving high atrial pressure. The two ventricles are inferior to the atria and they form the largest portion of the heart. Their walls are significantly thicker than those of the atria as a large amount of muscle mass is needed to push the blood into the arteries. The right ventricle makes up the right side and much of the anterior portion of the heart, while the left ventricle makes up the left side and much of the posterior portion of the heart (Figure \(\PageIndex{6}\)).
Also prominent along the superficial surfaces of the heart is a series of fat-filled grooves, each of which is known as a sulcus (plural = sulci), containing major coronary blood vessels. The deepest is the coronary sulcus, which encircles the heart between the atria and ventricles. Located between the left and right ventricles are two shallower sulci: the anterior interventricular sulcus is visible on the anterior surface of the heart (Figure \(\PageIndex{8.A}\)), whereas the posterior interventricular sulcus is visible on the posterior surface of the heart (Figure \(\PageIndex{8.B}\)).
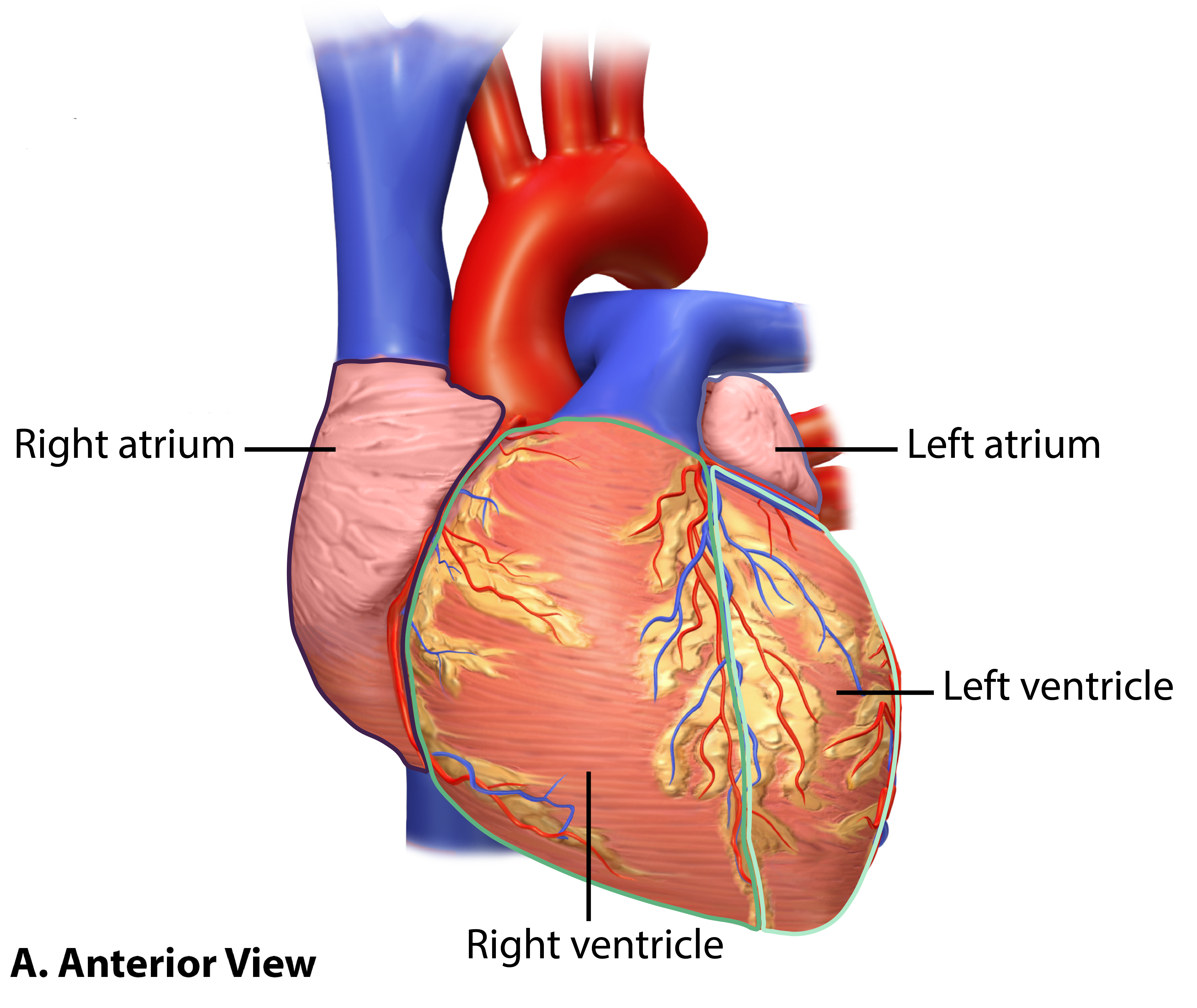
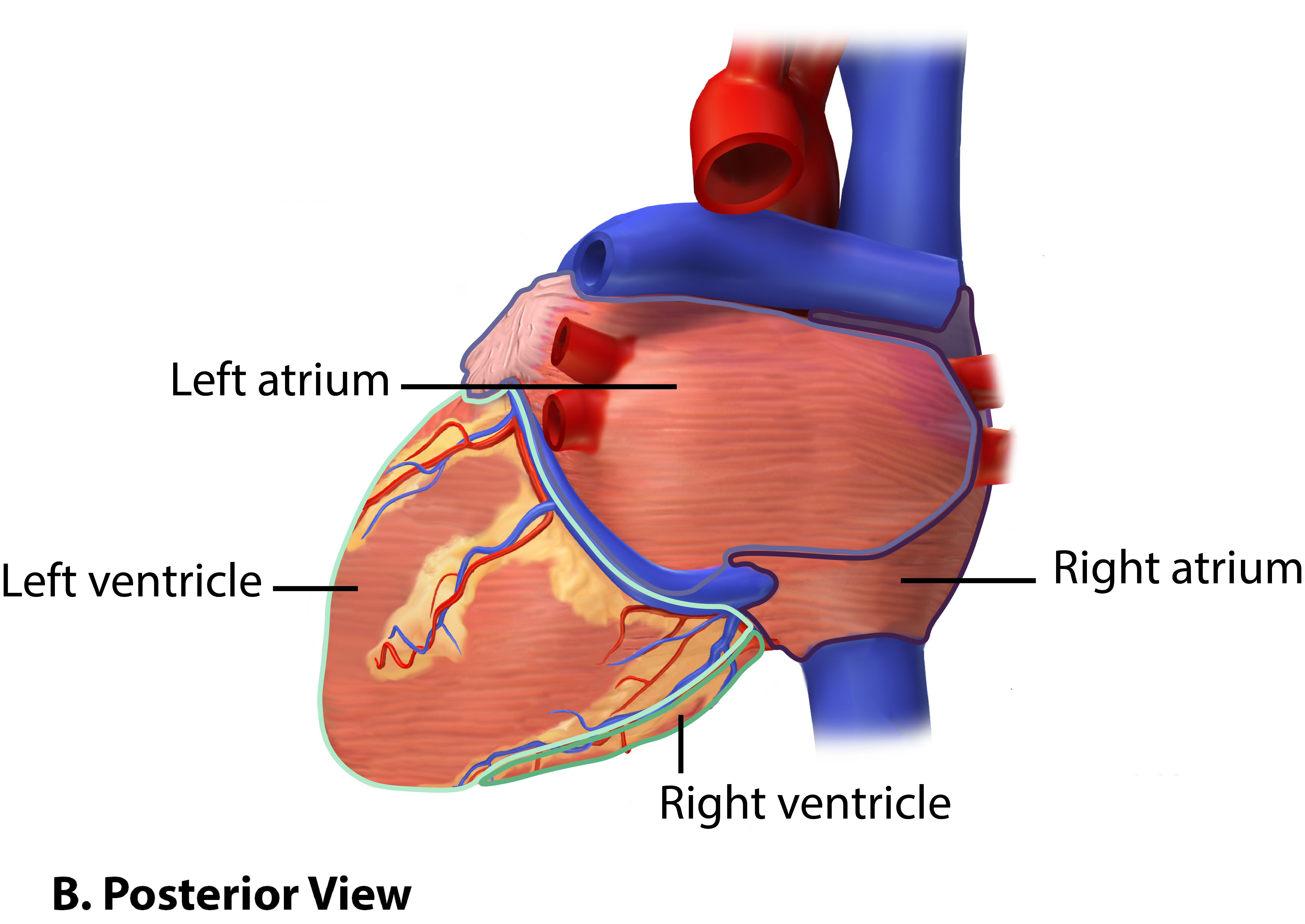
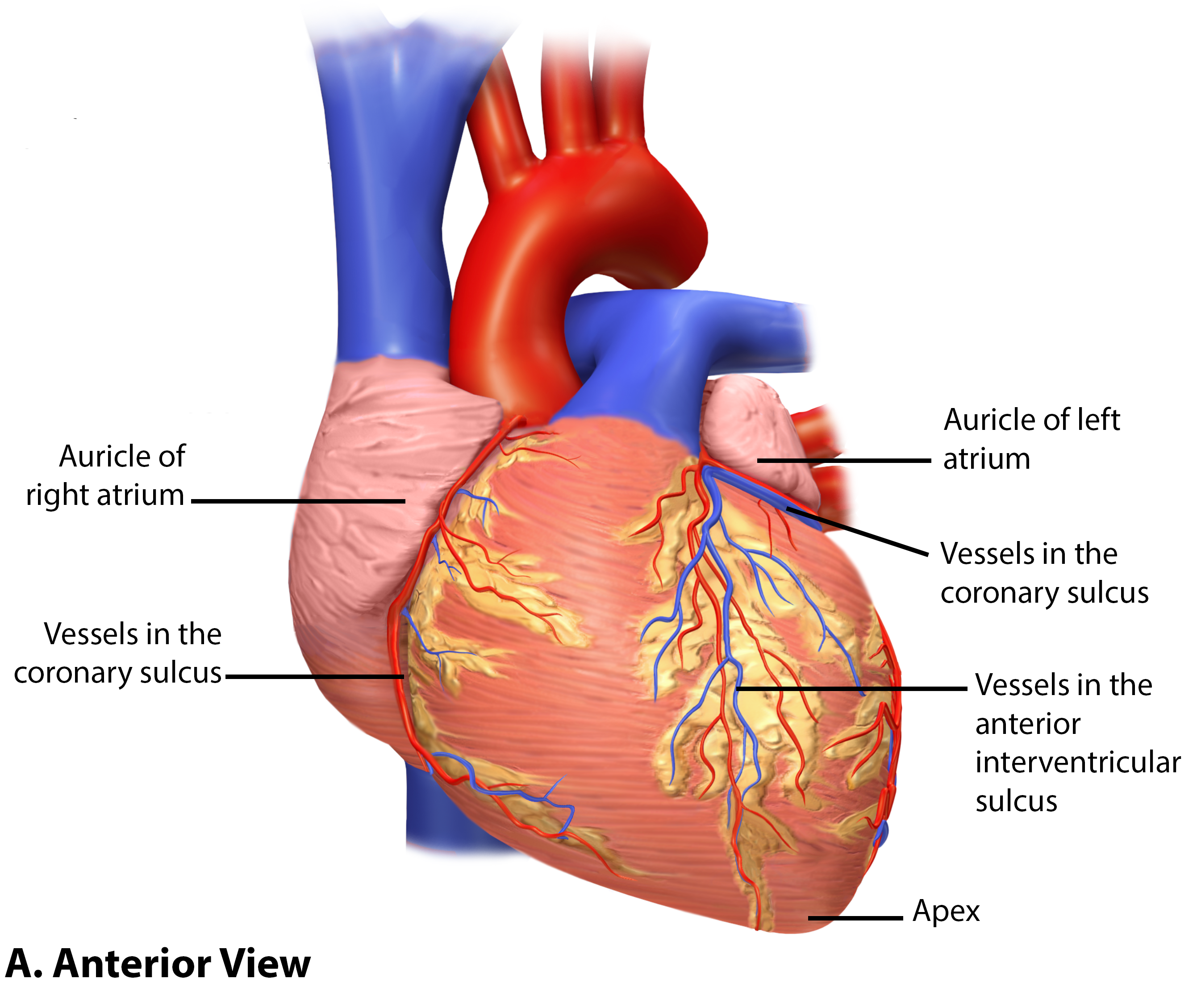
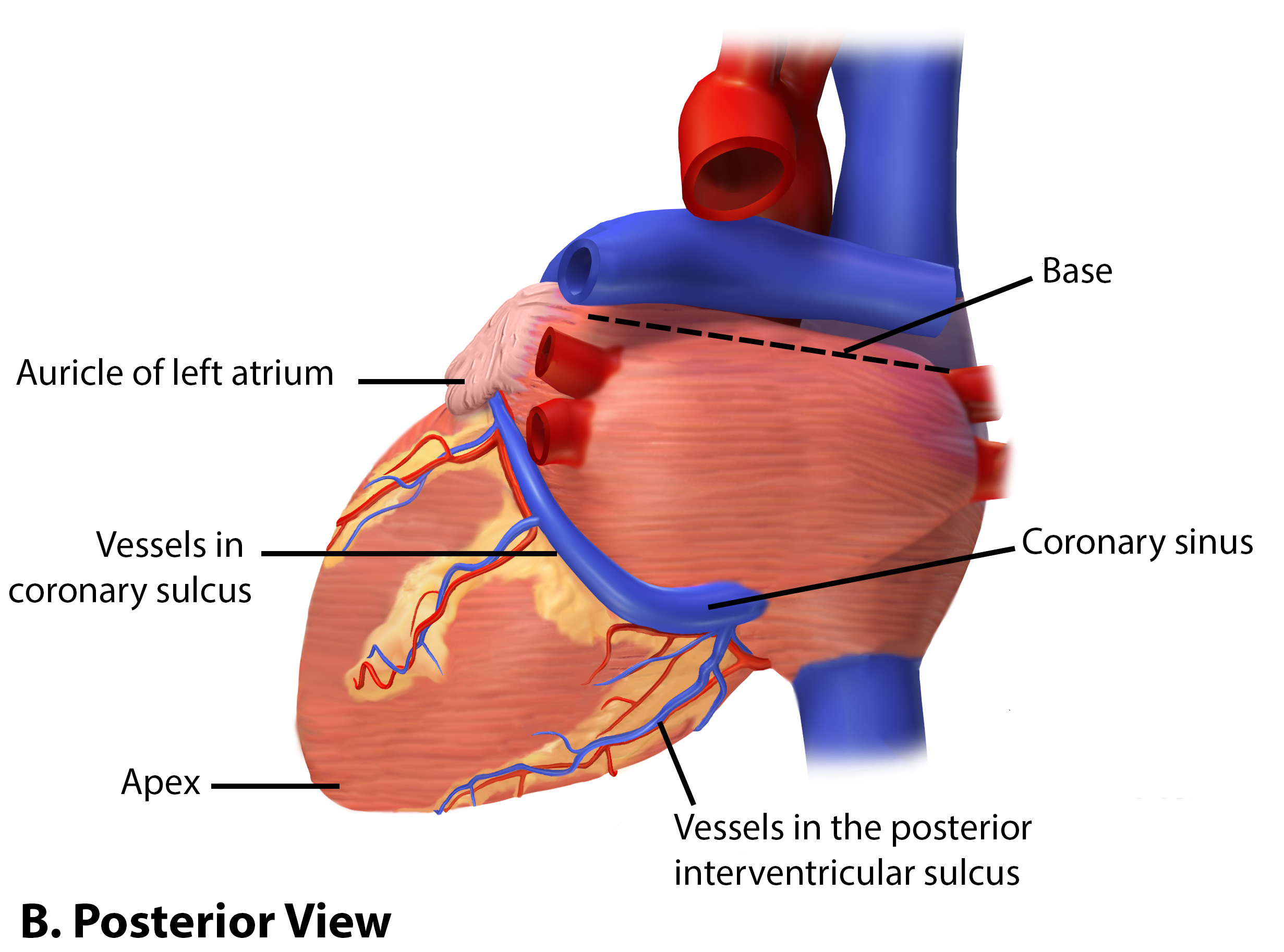
The Great Vessels
Blood enters and exits the heart through the largest arteries and veins, respectively, in the body. Termed the great vessels, these are the large arteries and veins directly connected with the heart, the: inferior vena cava, superior vena cava, pulmonary arteries, pulmonary veins, and the aorta (Figure \(\PageIndex{9}\)).
The pulmonary trunk arises from the right ventricle and then splits into the right and left pulmonary arteries, each of which carries blood to the lung on their respective side. After circulating through the smaller blood vessels of the lungs the blood, which will have a relatively high oxygenation level and a relatively low carbon dioxide level, returns to the heart in the right and left pulmonary veins and enters the left atrium and then the left ventricle. The left side of the heart gives rise to the largest and thickest artery in the body, the aorta. The aorta directs blood along multiple systemic circuit routes to reach all of the body's organs. The systemic circuit returns blood to the right atrium through two of the great vessels. The inferior vena cava carries blood from regions inferior to the heart while the superior vena cava collects blood from the regions superior to the heart. The structure of these vessels will be discussed in Chapter 18.
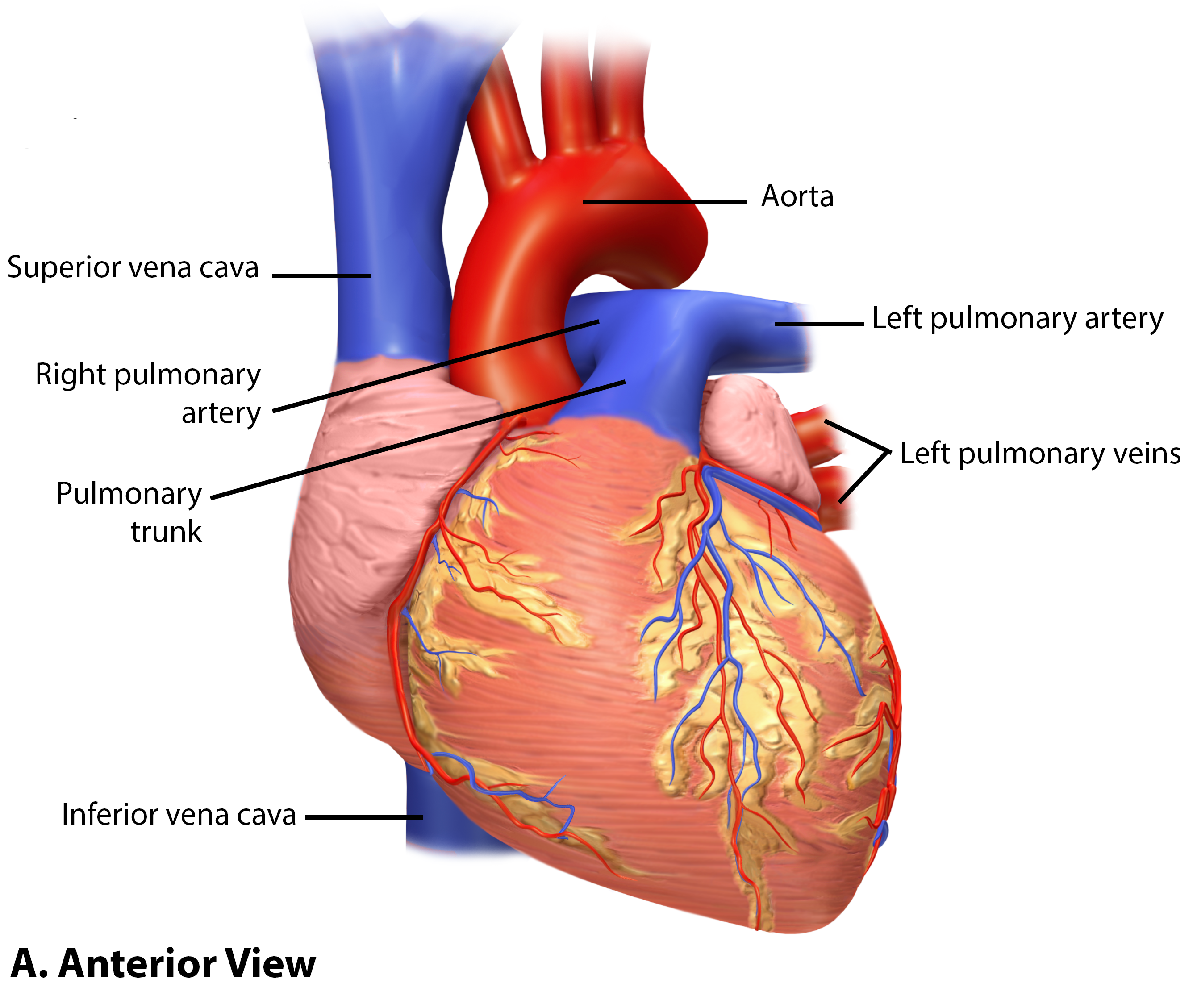
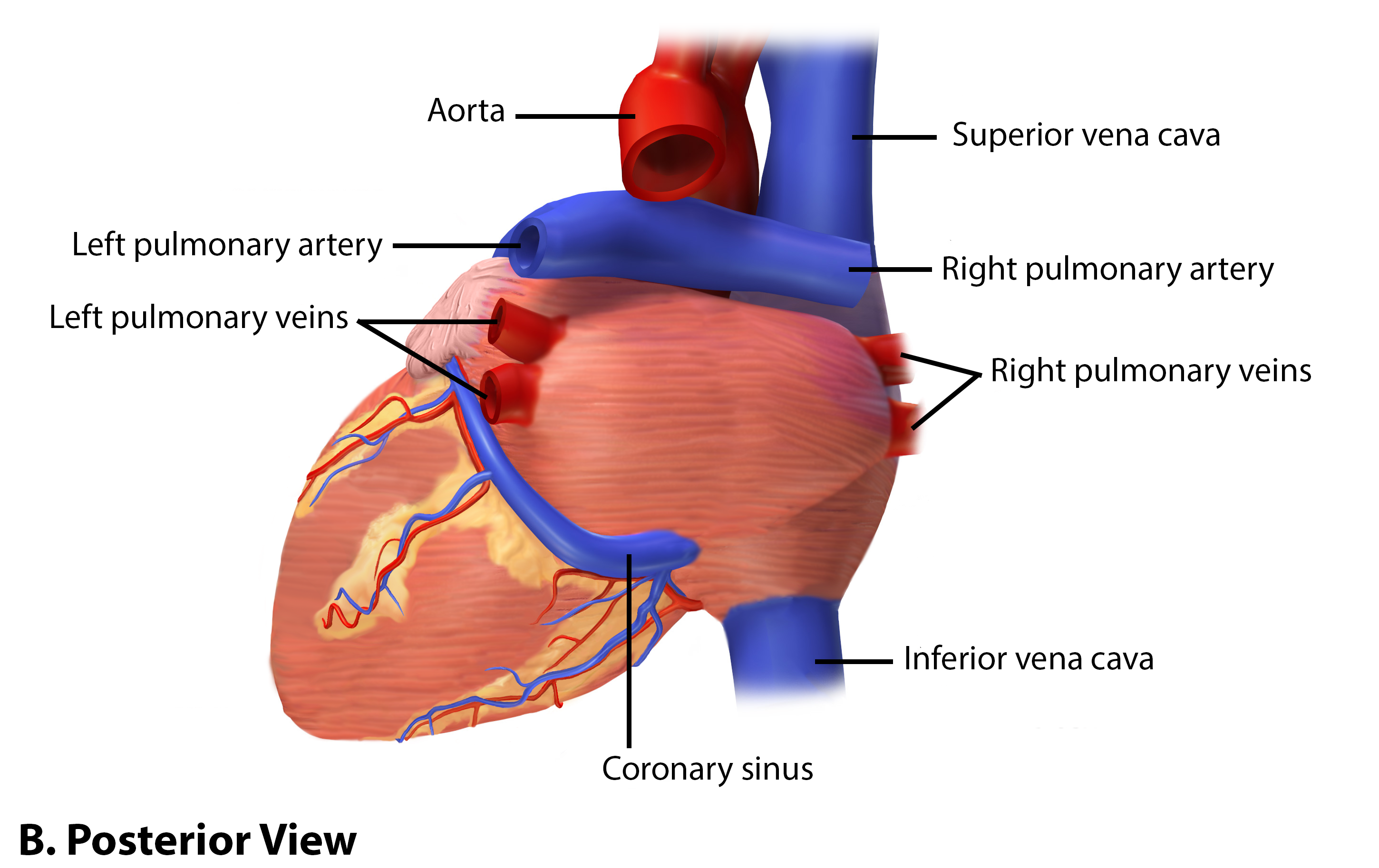
Explore a model of the heart in 3D:
Internal Structures of the Heart
Figure \(\PageIndex{10}\) shows the internal anatomy of the heart and the relative positions and features of the four chambers. Muscular septa form the walls between adjacent chambers. The word septum is derived from the Latin for “something that encloses;” in this case, a septum (plural = septa) refers to a wall or partition that divides the heart into chambers. The septa are physical extensions of the myocardium lined with endocardium. Located between the two atria is the interatrial septum. Normally in an adult heart, the interatrial septum bears an oval-shaped depression known as the fossa ovalis, a remnant of an opening in the fetal heart known as the foramen ovale. The foramen ovale allowed blood in the fetal heart to pass directly from the right atrium to the left atrium, allowing some blood to bypass the pulmonary circuit. Within seconds after birth, a flap of tissue that previously acted as a valve closes the foramen ovale and establishes the typical cardiac circulation pattern. Between the two ventricles is a second septum known as the interventricular septum. Unlike the interatrial septum, the interventricular septum is normally intact after its formation during fetal development. It is substantially thicker than the interatrial septum, since the ventricles generate far greater pressure when they contract.
The septum between the atria and ventricles is known as the atrioventricular septum. It is marked by the presence of four openings that allow blood to move from the atria into the ventricles and from the ventricles into the pulmonary trunk and aorta. Located in each of these openings between the atria and ventricles is a valve, a specialized structure that ensures one-way flow of blood. The valves between the atria and ventricles are known generically as atrioventricular valves. Each flap of an atrioventricular valve is attached to strong strands of connective tissue, the chordae tendineae, literally “tendinous cords,” or sometimes more poetically referred to as “heart strings” (Figure \(\PageIndex{11}\)). There are several chordae tendineae associated with each of the valve flaps that are composed of approximately 80 percent collagenous fibers with the remainder consisting of elastic fibers and endothelium. They connect each of the flaps to a papillary muscle. The valves at the openings that lead to the pulmonary trunk and aorta are known generically as semilunar valves. The semilunar valves are both comprised of three small flaps of endothelium reinforced with dense connective tissue.
Right Atrium
The right atrium serves as the receiving chamber for blood returning to the heart from the systemic circulation. The two major systemic veins, the superior and inferior venae cavae, and the large coronary vein called the coronary sinus empty into the right atrium. The superior vena cava drains blood from regions superior to the diaphragm: the head, neck, upper limbs, and the thoracic region. It empties into the superior and posterior portions of the right atrium. The inferior vena cava drains blood from areas inferior to the diaphragm: the lower limbs and abdominopelvic region of the body. It, too, empties into the posterior portion of the atria, but inferior to the opening of the superior vena cava. Immediately superior and slightly medial to the opening of the inferior vena cava on the posterior surface of the atrium is the opening of the coronary sinus. This thin-walled vessel drains most of the coronary veins that return systemic blood from the heart into the right atrium. The majority of the internal heart structures discussed in this and subsequent sections are illustrated in Figure \(\PageIndex{10}\).
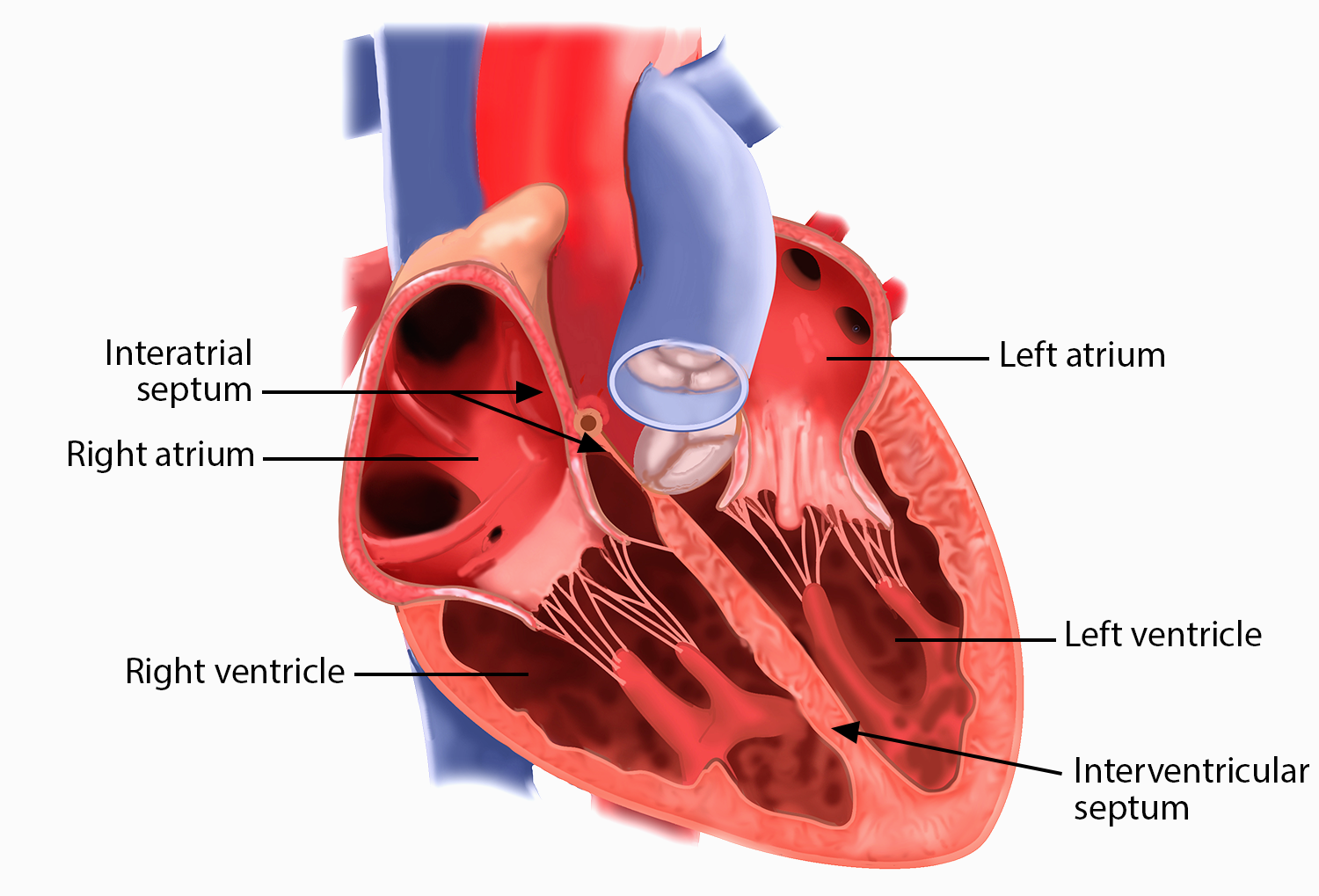
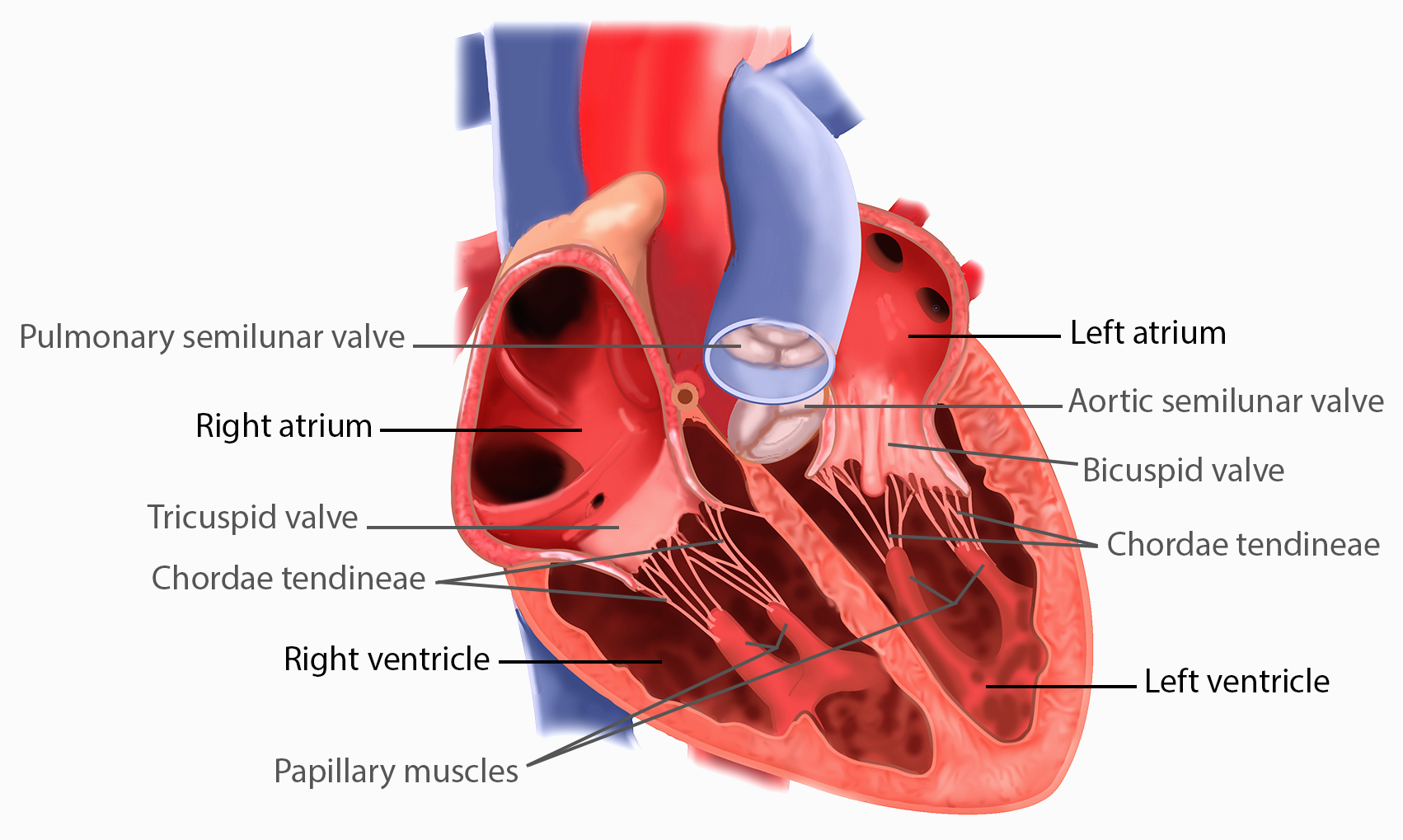
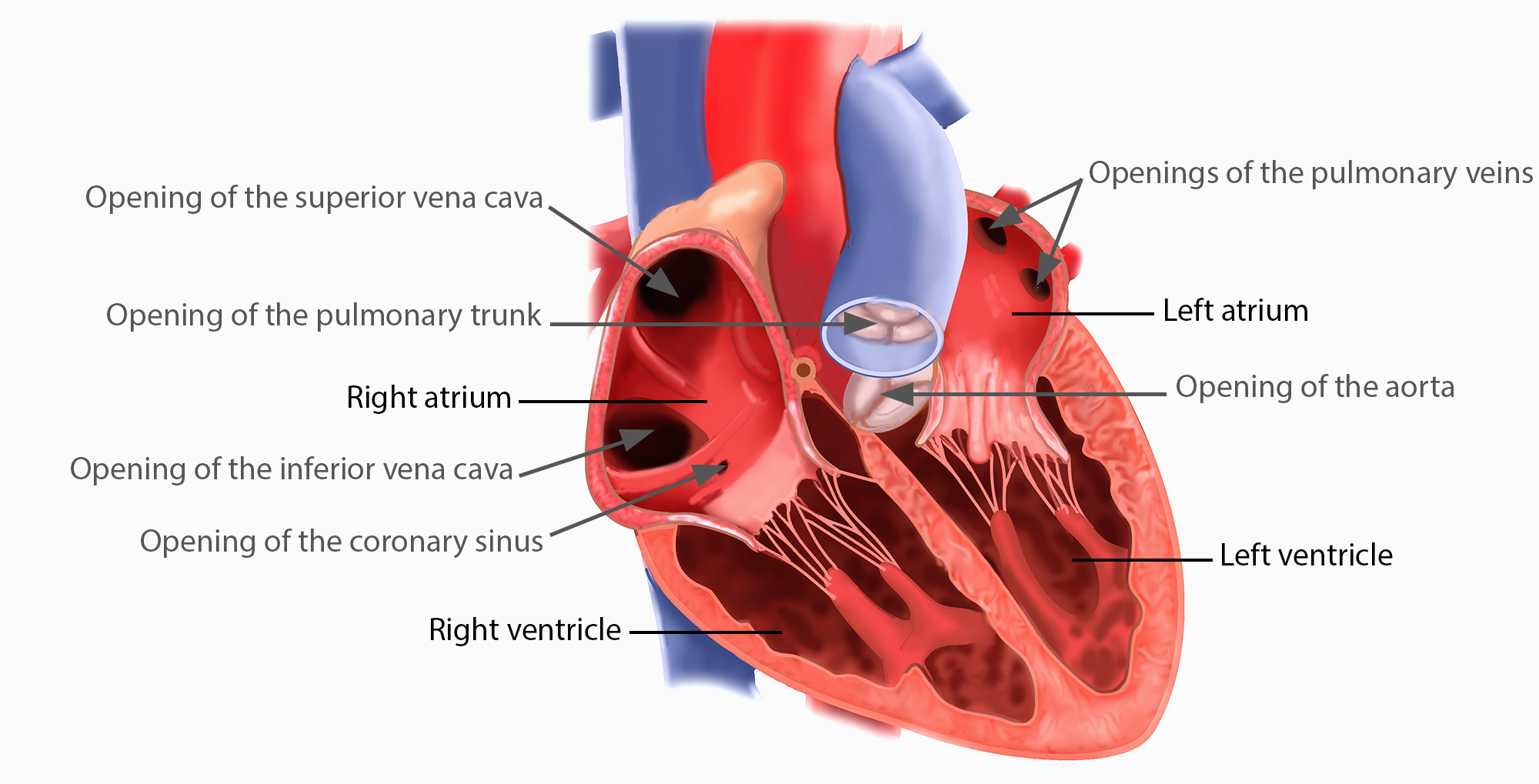
While the bulk of the internal surface of the right atrium is smooth, the depression of the fossa ovalis is medial, and the anterior surface demonstrates prominent ridges of muscle called the pectinate muscles (see Figure \(\PageIndex{11}\)), which are thought to help spread the electrical signals to contract and strengthen the contraction of the atria. The right auricle also has pectinate muscles. The left atrium does not have pectinate muscles except in the auricle.
The atria receive venous blood on a nearly continuous basis, allowing the heart to continue to receive blood even while the ventricles are contracting. While most ventricular filling occurs while the atria are relaxed, they do demonstrate a contractile phase and actively pump blood into the ventricles just prior to ventricular contraction. The opening between the right atrium and right ventricle is guarded by the tricuspid valve.
Right Ventricle
The right ventricle receives blood from the right atrium through the tricuspid valve (also called the right atrioventricular valve). There are three papillary muscles in the right ventricle which correspond to the three flaps of the valve. (The third valve flap and papillary muscle would be on the anterior portion of the heart, which has been removed in this illustration.) When the myocardium of the ventricle contracts, pressure within the ventricular chamber rises. Blood, like any fluid, flows from higher pressure to lower pressure areas, in this case, toward the pulmonary trunk and the atrium. To prevent any potential backflow, the flaps of the tricuspid valve are pushed closed during ventricular contraction. The papillary muscles contract with the ventricular myocardium, generating tension on the chordae tendineae. This prevents the flaps of the valves from being forced into the atria and regurgitation of the blood back into the atria during ventricular contraction. Figure \(\PageIndex{11}\) shows papillary muscles and chordae tendineae attached to the bicuspid valve.
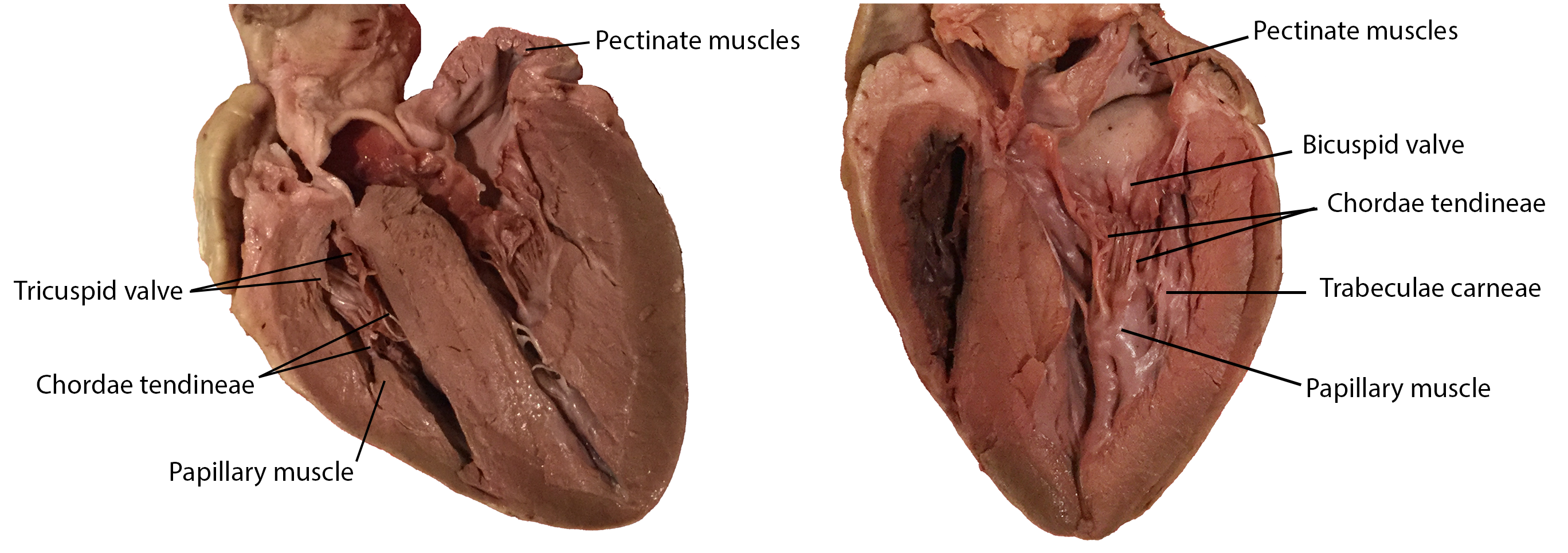
The walls of the ventricle are lined with trabeculae carneae, ridges of cardiac muscle covered by endocardium (Figure \(\PageIndex{11}\)) that increase the surface area of the ventricular wall.
When the right ventricle contracts, it ejects blood into the pulmonary trunk, which branches into the left and right pulmonary arteries that carry deoxygenated blood to each lung. The superior surface of the right ventricle begins to taper as it approaches the pulmonary trunk. At the base of the pulmonary trunk is the pulmonary semilunar valve that prevents backflow from the pulmonary trunk when the ventricle relaxes.
Left Atrium
After exchange of gases in the pulmonary capillaries, freshly oxygenated blood returns to the left atrium via one of the four pulmonary veins. The left atrium does not contain pectinate muscles so its walls are smoother than in the right atrium, but it does have an auricle that includes pectinate ridges. Blood flows nearly continuously from the pulmonary veins back into the atrium, which acts as the receiving chamber, and from here through an open bicuspid valve, also known as the mitral valve or the left atrioventricular valve, into the left ventricle. Most blood flows passively into the heart while both the atria and ventricles are relaxed, but toward the end of the ventricular relaxation period, the left atrium will contract, pumping blood into the ventricle. This atrial contraction accounts for approximately 20 percent of ventricular filling.
Left Ventricle
Recall that, although both sides of the heart will pump the same amount of blood, the muscular layer is much thicker in the left ventricle compared to the right (see Figure \(\PageIndex{7}\)). The left ventricle is the major pumping chamber for the systemic circuit; it ejects blood into the aorta through the aortic semilunar valve. The primary driver of the myocardial thickness of the left ventricle is the need to push blood into the head to ensure the brain has a constant supply - this requires more force because of the larger distance and the resistance of gravity.
The left ventricle also has trabeculae carneae, and they are more prominent here than in the right ventricle. The flaps of the bicuspid valve are connected to papillary muscles via chordae tendineae. There are two papillary muscles in the left ventricle—the anterior and posterior—as opposed to three in the right ventricle.
Heart Valve Function
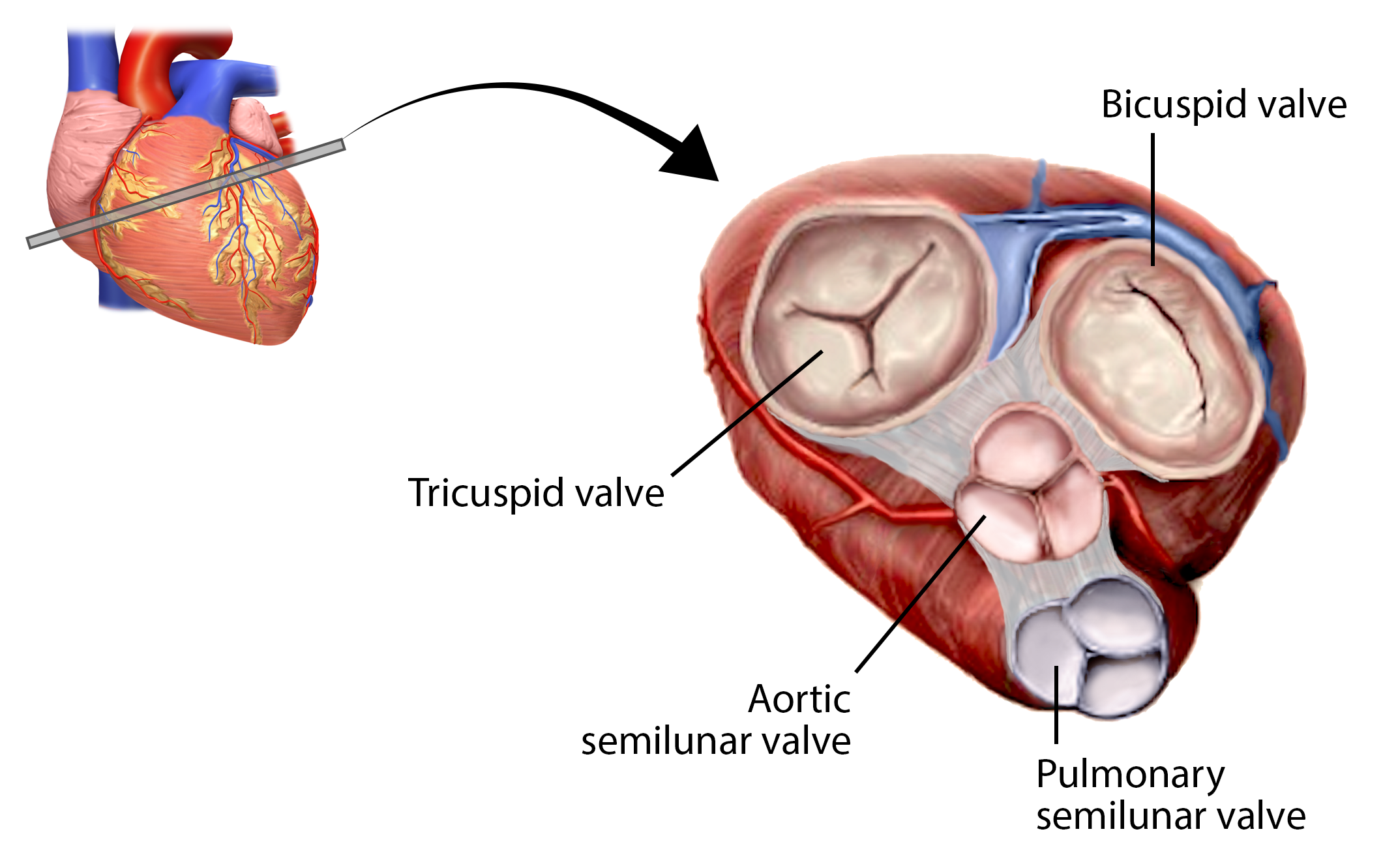
A transverse section through the heart slightly above the level of the atrioventricular septum reveals all four heart valves along the same plane (Figure \(\PageIndex{12}\)). The valves ensure unidirectional blood flow through the heart. Between the right atrium and the right ventricle is the right atrioventricular valve, or tricuspid valve. It typically consists of three flaps, or leaflets, made of endocardium reinforced with additional dense connective tissue. Each flap is connected by several chordae tendineae to a papillary muscle that protrudes from the ventricular wall.
Located at the opening between the left atrium and left ventricle is the mitral valve, also called the bicuspid valve or the left atrioventricular valve. In a clinical setting, the valve is referred to as the mitral valve, rather than the bicuspid valve, as the actual number of cusps has been found to vary greatly among individuals (Gunnal et al, 2012). The cusps of the mitral valve are attached by chordae tendineae to two papillary muscles that project from the trabeculae carneae of the wall of the ventricle.
When the ventricles begin to contract, pressure within the ventricles rises and blood flows toward the area of lowest pressure, which is initially in the atria. This movement of blood causes the cusps of the tricuspid and mitral valves to close. As the myocardium of the ventricle contracts, so do the papillary muscles. This creates tension on the chordae tendineae (see Figure \(\PageIndex{13}\) - ventricular systole), helping to hold the cusps of the atrioventricular valves in place and preventing them from everting into the atria. During the relaxation phase of the cardiac cycle, the papillary muscles are also relaxed and the tension on the chordae tendineae is slight.
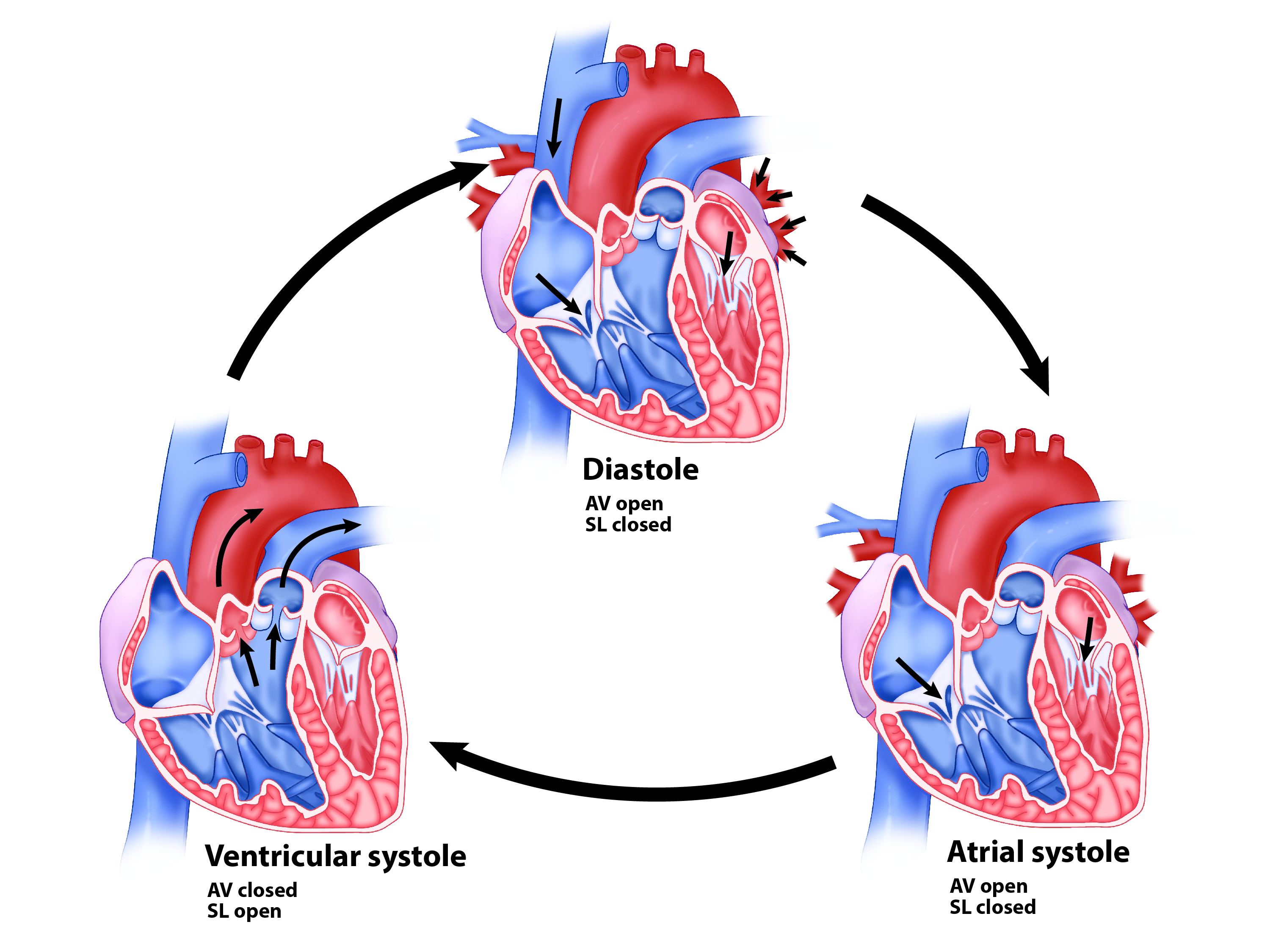
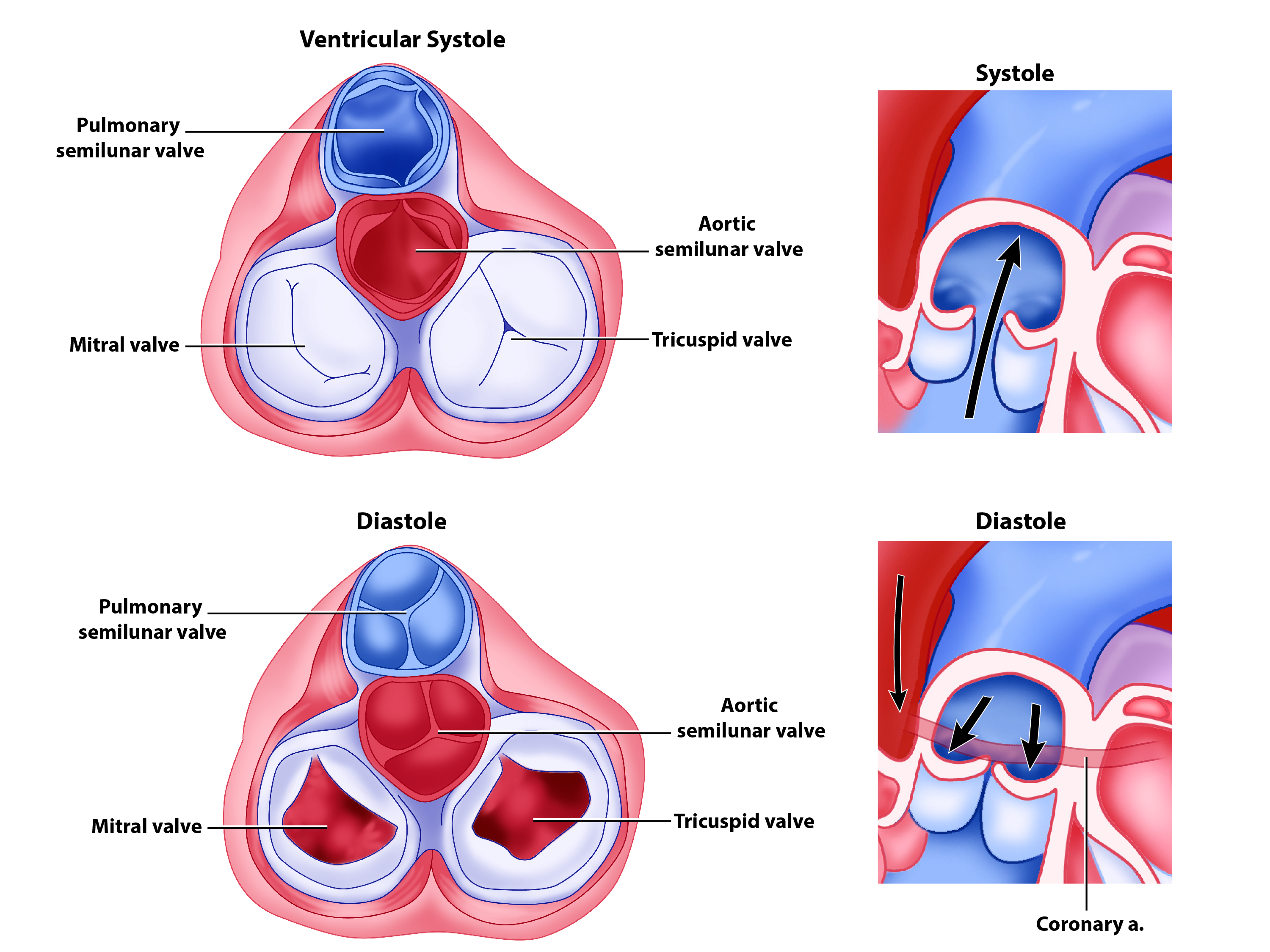
Emerging from the right ventricle at the base of the pulmonary trunk is the pulmonary semilunar valve, or the pulmonary valve; it is also known as the pulmonic valve. At the base of the aorta that emerges from the left ventricle is the aortic semilunar valve, or the aortic valve. The semilunar valves are both comprised of three small flaps of endothelium reinforced with dense connective tissue.
When the ventricle relaxes, the pressure differential causes blood to flow back towards the ventricle within the pulmonary trunk and aorta. This flow of blood fills the pocket-like flaps of each semilunar valve, causing the valve to close, disrupting the backflow of blood and producing an audible sound. Unlike the atrioventricular valves, there are no papillary muscles or chordae tendineae associated with the semilunar valves.
Since these openings and valves structurally weaken the atrioventricular septum, the remaining tissue is heavily reinforced with dense irregular connective tissue of the cardiac skeleton, or skeleton of the heart. It includes four rings that surround the openings between the atria and ventricles, and the openings to the pulmonary trunk and aorta, and serve as the point of attachment for the heart valves. The cardiac skeleton also provides the structural framework against which the cardiac muscle contracts and forms an important insulating boundary in the heart's electrical conducting system.
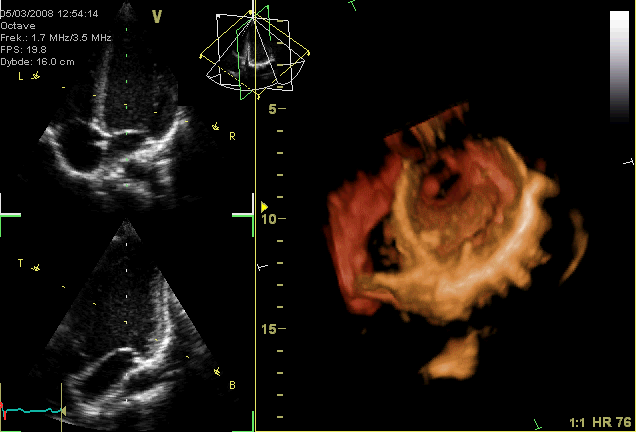
Although much of the heart has been “removed” from this gif loop so the chordae tendineae are not visible, why is their presence more critical for the atrioventricular valves (tricuspid and mitral) than the semilunar (aortic and pulmonary) valves?
-
Answer
-
The pressure gradient between the atria and the ventricles is much greater than that between the ventricles and the pulmonary trunk and aorta. Without the presence of the chordae tendineae and papillary muscles, the valves would be blown back (prolapsed) into the atria and blood would regurgitate.
Valves and Heart Sounds
In a normal, healthy heart, there are primarily two audible heart sounds: S1 and S2. S1 is the sound created by the closing of the atrioventricular valves during ventricular contraction and is normally described as a “lub,” or first heart sound. The second heart sound, S2, is the sound of the closing of the semilunar valves during ventricular diastole and is described as a “dub”. In both cases, as the valves close, the openings within the atrioventricular septum guarded by the valves will become reduced, and blood flow through the opening will become more turbulent until the valves are fully closed. There is a third heart sound, S3, but it is rarely heard in healthy individuals.
The term murmur is used to describe an unusual sound coming from the heart that is caused by the turbulent flow of blood. Murmurs are graded on a scale of 1 to 6, with 1 being the most common, the most difficult sound to detect, and the least serious. Placement of the stethoscope in four distinct locations enables optimal ausculation (listening with a stethoscope) of each valve: near transition of the ascending aorta to the aortic arch for the aortic valve, near the bifurcation of the pulmonary trunk for the pulmonary valve, near the superior portion of the right ventricle for the tricuspid valve, and near the apex of the heart for the bicuspid valve. Figure \(\PageIndex{15}\) indicates proper placement of the bell of the stethoscope to facilitate auscultation.
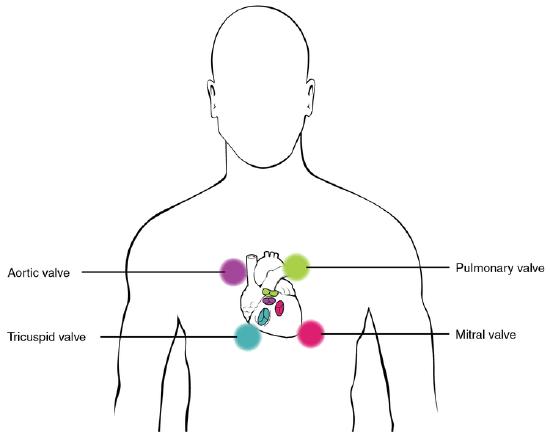
Heart Valves
When heart valves do not function properly, they are often described as incompetent and result in valvular heart disease, which can range from benign to lethal. Some of these conditions are congenital, that is, the individual was born with the defect, whereas others may be attributed to disease processes or trauma. Some malfunctions are treated with medications, others require surgery, and still others may be mild enough that the condition is merely monitored since treatment might trigger more serious consequences.
Valvular disorders are often caused by carditis, or inflammation of the heart. One common trigger for this inflammation is rheumatic fever, or scarlet fever, an autoimmune response to the presence of a bacterium, Streptococcus pyogenes, normally a disease of childhood.
While any of the heart valves may be involved in valve disorders, bicuspid regurgitation is the most common, detected in approximately 2 percent of the population, and the pulmonary semilunar valve is the least frequently involved. When a valve malfunctions, the flow of blood to a region will often be disrupted. The resulting inadequate flow of blood to this region will be described in general terms as an insufficiency. The specific type of insufficiency is named for the valve involved: aortic insufficiency, mitral insufficiency, tricuspid insufficiency, or pulmonary insufficiency.
If one of the cusps of the valve is forced backward by the force of the blood, the condition is referred to as a prolapsed valve. Prolapse may occur if the chordae tendineae are damaged or broken, causing the closure mechanism to fail. The failure of the valve to close properly disrupts the normal one-way flow of blood and results in regurgitation, when the blood flows backward from its normal path. Using a stethoscope, the disruption to the normal flow of blood produces a heart murmur.
Stenosis is a condition in which the heart valves become rigid and may calcify over time. The loss of flexibility of the valve interferes with normal function and may cause the heart to work harder to propel blood through the valve, which eventually weakens the heart. Aortic stenosis affects approximately 2 percent of the population over 65 years of age, and the percentage increases to approximately 4 percent in individuals over 85 years. Occasionally, one or more of the chordae tendineae will tear or the papillary muscle itself may die as a component of a myocardial infarction (heart attack). In this case, the patient’s condition will deteriorate dramatically and rapidly, and immediate surgical intervention may be required.
Auscultation, or listening to a patient’s heart sounds, is one of the most useful diagnostic tools, since it is proven, safe, and inexpensive. The term auscultation is derived from the Latin for “to listen,” and the technique has been used for diagnostic purposes as far back as the ancient Egyptians. Valve and septal disorders will trigger abnormal heart sounds. If a valvular disorder is detected or suspected, a test called an echocardiogram, or simply an “echo,” may be ordered. Echocardiograms are sonograms of the heart and can help in the diagnosis of valve disorders as well as a wide variety of heart pathologies.
Circulation Through the Heart
The right ventricle pumps deoxygenated blood into the pulmonary trunk, which ascends across the anterior surfaces of the ascending aorta and left atrium toward a bifurcation into the left and right pulmonary arteries. The right pulmonary artery passes under the aortic arch and delivers blood to the right lung. The left pulmonary artery delivers blood to the left lung. These arteries in turn branch many times in each lung before reaching the pulmonary capillaries, where gas exchange occurs: carbon dioxide exits the blood and oxygen enters. The pulmonary trunk, arteries, and their branches are the only arteries in the post-natal body that carry relatively deoxygenated blood. Highly oxygenated blood returning from the pulmonary capillaries in the lungs passes through a series of vessels that join together to form the pulmonary veins—the only post-natal veins in the body that carry highly oxygenated blood. The pulmonary veins conduct blood into the right and left sides of the left atrium posteriorly, which pumps the blood into the left ventricle, which in turn pumps oxygenated blood into the aorta, which ascends out of the left ventricle posterior to the pulmonary trunk, arches over the top of the heart and descends posterior to the heart. The aorta branches to deliver oxygenated blood throughout the body via the systemic circuit. Eventually, blood reaches systemic capillaries, where exchange with the tissue fluid and cells of the body occurs. In this case, oxygen and nutrients exit the systemic capillaries to be used by the cells in their metabolic processes, and carbon dioxide and waste products will enter the blood.
The blood exiting the systemic capillaries is lower in oxygen concentration than when it entered. The capillaries will ultimately unite to form venules, joining to form ever-larger veins, eventually flowing into the two major systemic veins, the superior vena cava and the inferior vena cava, which return blood into the right atrium. The blood in the superior and inferior venae cavae flows into the right atrium, which pumps blood into the right ventricle (Figure \(\PageIndex{4}\)).
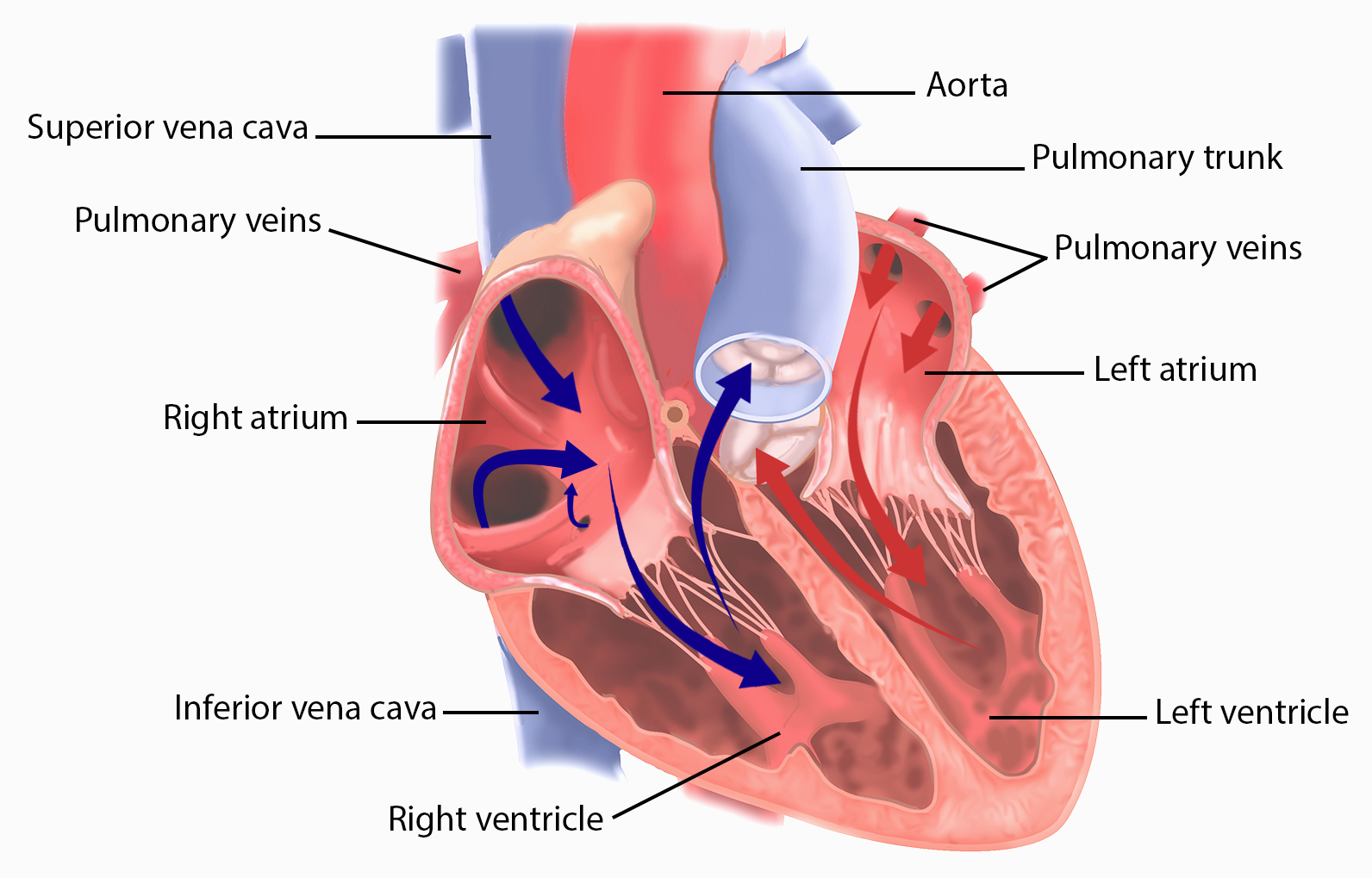
Cardiovascular Technologist/Technician
Cardiovascular technologists/technicians are trained professionals who perform a variety of imaging techniques, such as sonograms or echocardiograms, used by physicians to diagnose and treat diseases of the heart. Nearly all of these positions require an associate degree, and these technicians earn a median salary of $68,750 as of 2019. Growth within the field is fast, projected at 12 percent from 2019 - 2029, according to the U.S. Bureau of Labor Statistics.
Visit this site for more information on cardiovascular technologists/technicians [statistics retrieved 10 Dec 2020].
There is a considerable overlap and complementary skills between cardiac technicians and vascular technicians, and so the term cardiovascular technician is often used. Special certifications within the field require documenting appropriate experience and completing additional and often expensive certification examinations. These subspecialties include Certified Rhythm Analysis Technician (CRAT), Certified Cardiographic Technician (CCT), Registered Congenital Cardiac Sonographer (RCCS), Registered Cardiac Electrophysiology Specialist (RCES), Registered Cardiovascular Invasive Specialist (RCIS), Registered Cardiac Sonographer (RCS), Registered Vascular Specialist (RVS), and Registered Phlebology Sonographer (RPhS).
Concept Review
The heart resides within the pericardial sac and is located in the mediastinal space within the thoracic cavity. The pericardial sac consists of two fused layers: an outer fibrous layer and an inner parietal pericardial serous membrane. Between the pericardial sac and the heart is the pericardial cavity, which is filled with lubricating serous fluid. The walls of the heart are composed of an outer epicardium, a thick myocardium, and an inner lining layer of endocardium. The human heart consists of a pair of atria, which receive blood and pump it into a pair of ventricles, which pump blood into the vessels. The right atrium receives systemic blood relatively low in oxygen and pumps it into the right ventricle, which pumps it into the pulmonary circuit. Exchange of oxygen and carbon dioxide occurs in the lungs, and blood high in oxygen returns to the left atrium, which pumps blood into the left ventricle, which in turn pumps blood into the aorta and the remainder of the systemic circuit. The septa are the partitions that separate the chambers of the heart. They include the interatrial septum, the interventricular septum, and the atrioventricular septum. Two of these openings are guarded by the atrioventricular valves, the right tricuspid valve and the left mitral valve, which prevent the backflow of blood. Each is attached to chordae tendineae that extend to the papillary muscles, which are extensions of the myocardium, to prevent the valves from being blown back into the atria. The pulmonary semilunar valve is located at the base of the pulmonary trunk, and the aortic semilunar valve is located at the base of the aorta.
Review Questions
Q. Which of the following is not important in preventing backflow of blood?
A. chordae tendineae
B. papillary muscles
C. AV valves
D. myocardium
- Answer
-
C
Q. Which valve separates the left atrium from the left ventricle?
A. mitral
B. tricuspid
C. pulmonary
D. aortic
- Answer
-
A
Q. Which of the following lists the valves in the order through which the blood flows from the vena cava through the heart?
A. tricuspid, pulmonary semilunar, bicuspid, aortic semilunar
B. mitral, pulmonary semilunar, bicuspid, aortic semilunar
C. aortic semilunar, pulmonary semilunar, tricuspid, bicuspid
D. bicuspid, aortic semilunar, tricuspid, pulmonary semilunar
- Answer
-
A
Q. Which chamber initially receives blood from the systemic circuit?
A. left atrium
B. left ventricle
C. right atrium
D. right ventricle
- Answer
-
C
Q. The myocardium would be the thickest in the ________.
A. left atrium
B. left ventricle
C. right atrium
D. right ventricle
- Answer
-
B
Q. Most blood enters the ventricle during ________.
A. atrial systole
B. atrial diastole
C. ventricular systole
D. cardiac diastole
- Answer
-
D
Q. The first heart sound represents which portion of the cardiac cycle?
A. atrial systole
B. ventricular systole
C. closing of the atrioventricular valves
D. closing of the semilunar valves
- Answer
-
Answer: C
Q. In which septum is it normal to find openings in the adult?
A. interatrial septum
B. interventricular septum
C. atrioventricular septum
D. all of the above
- Answer
-
C
Critical Thinking Questions
Q. Describe the cardiac cycle, beginning with both atria and ventricles relaxed.
- Answer
-
A. The cardiac cycle comprises a complete relaxation and contraction of both the atria and ventricles, and lasts approximately 0.8 seconds. Beginning with all chambers in diastole, blood flows passively from the veins into the atria and past the atrioventricular valves into the ventricles. The atria begin to contract following depolarization of the atria and pump blood into the ventricles. The ventricles begin to contract, raising pressure within the ventricles. When ventricular pressure rises above the pressure in the two major arteries, blood pushes open the two semilunar valves and moves into the pulmonary trunk and aorta in the ventricular ejection phase. Following ventricular repolarization, the ventricles begin to relax, and pressure within the ventricles drops. When the pressure falls below that of the atria, blood moves from the atria into the ventricles, opening the atrioventricular valves and marking one complete heart cycle.
References
Axel L. “Papillary muscles do not attach directly to the solid heart wall.” Circulation. 2004 Jun 29;109(25):3145-8. doi: 10.1161/01.CIR.0000134276.06719.F3 [accessed 3 April 2021].
Gunnal, S A et al. “Study of mitral valve in human cadaveric hearts.” Heart views: the official journal of the Gulf Heart Association vol. 13,4 (2012): 132-5. doi:10.4103/1995-705X.105729 [accessed 3 April 2021].
Popa-Fotea, Nicoleta Monica et al. “Exploring the Continuum of Hypertrophic Cardiomyopathy-From DNA to Clinical Expression.” Medicina (Kaunas, Lithuania) vol. 55,6 299. 23 Jun. 2019, doi:10.3390/medicina55060299 [accessed 2 April 2021].
Glossary
- anterior interventricular sulcus
- sulcus located between the left and right ventricles on the anterior surface of the heart
- aortic valve
- (also, aortic semilunar valve) valve located at the base of the aorta
- atrioventricular septum
- cardiac septum located between the atria and ventricles; atrioventricular valves are located here
- atrioventricular valves
- one-way valves located between the atria and ventricles; the valve on the right is called the tricuspid valve, and the one on the left is the mitral or bicuspid valve
- atrium
- (plural = atria) upper or receiving chamber of the heart that pumps blood into the lower chambers just prior to their contraction; the right atrium receives blood from the systemic circuit that flows into the right ventricle; the left atrium receives blood from the pulmonary circuit that flows into the left ventricle
- auricle
- extension of an atrium visible on the anterosuperior surface of the heart
- bicuspid valve
- (also, mitral valve or left atrioventricular valve) valve located between the left atrium and ventricle; consists of two flaps of tissue
- cardiac notch
- depression in the medial surface of the inferior lobe of the left lung where the apex of the heart is located
- cardiac skeleton
- (also, skeleton of the heart) reinforced connective tissue located within the atrioventricular septum; includes four rings that surround the openings between the atria and ventricles, and the openings to the pulmonary trunk and aorta; the point of attachment for the heart valves
- chordae tendineae
- string-like extensions of tough connective tissue that extend from the flaps of the atrioventricular valves to the papillary muscles
- coronary arteries
- branches of the ascending aorta that supply blood to the heart; the left coronary artery feeds the left side of the heart, the left atrium and ventricle, and the interventricular septum; the right coronary artery feeds the right atrium, portions of both ventricles, and the heart conduction system
- coronary sinus
- large, thin-walled vein on the posterior surface of the heart that lies within the atrioventricular sulcus and drains the heart myocardium directly into the right atrium
- coronary sulcus
- sulcus that marks the boundary between the atria and ventricles
- endocardium
- innermost layer of the heart lining the heart chambers and heart valves; composed of endothelium reinforced with a thin layer of connective tissue that binds to the myocardium
- endothelium
- layer of smooth, simple squamous epithelium that lines the endocardium and blood vessels
- epicardium
- innermost layer of the serous pericardium and the outermost layer of the heart wall
- foramen ovale
- opening in the fetal heart that allows blood to flow directly from the right atrium to the left atrium, bypassing the fetal pulmonary circuit
- fossa ovalis
- oval-shaped depression in the interatrial septum that marks the former location of the foramen ovale
- hypertrophic cardiomyopathy
- pathological enlargement of the heart, generally for no known reason
- inferior vena cava
- large systemic vein that returns blood to the heart from the inferior portion of the body
- interatrial septum
- cardiac septum located between the two atria; contains the fossa ovalis after birth
- interventricular septum
- cardiac septum located between the two ventricles
- mitral valve
- (also, left atrioventricular valve or bicuspid valve) valve located between the left atrium and ventricle; consists of two flaps of tissue
- mitral valve
- (also, left atrioventricular valve or bicuspid valve) valve located between the left atrium and ventricle; consists of two flaps of tissue
- myocardium
- thickest layer of the heart composed of cardiac muscle cells built upon a framework of primarily collagenous fibers and blood vessels that supply it and the nervous fibers that help to regulate it
- papillary muscle
- extension of the myocardium in the ventricles to which the chordae tendineae attach
- pectinate muscles
- muscular ridges seen on the anterior surface of the right atrium
- pericardial cavity
- cavity surrounding the heart filled with a lubricating serous fluid that reduces friction as the heart contracts
- pericardial sac
- (also, pericardium) membrane that separates the heart from other mediastinal structures; consists of two distinct, fused sublayers: the fibrous pericardium and the parietal pericardium
- pericardium
- (also, pericardial sac) membrane that separates the heart from other mediastinal structures; consists of two distinct, fused sublayers: the fibrous pericardium and the parietal pericardium
- posterior interventricular sulcus
- sulcus located between the left and right ventricles on the anterior surface of the heart
- pulmonary arteries
- left and right branches of the pulmonary trunk that carry deoxygenated blood from the heart to each of the lungs
- pulmonary capillaries
- capillaries surrounding the alveoli of the lungs where gas exchange occurs: carbon dioxide exits the blood and oxygen enters
- pulmonary circuit
- blood flow to and from the lungs
- pulmonary trunk
- large arterial vessel that carries blood ejected from the right ventricle; divides into the left and right pulmonary arteries
- pulmonary valve
- (also, pulmonary semilunar valve, the pulmonic valve, or the right semilunar valve) valve at the base of the pulmonary trunk that prevents backflow of blood into the right ventricle; consists of three flaps
- pulmonary veins
- veins that carry highly oxygenated blood into the left atrium, which pumps the blood into the left ventricle, which in turn pumps oxygenated blood into the aorta and to the many branches of the systemic circuit
- right atrioventricular valve
- (also, tricuspid valve) valve located between the right atrium and ventricle; consists of three flaps of tissue
- semilunar valves
- valves located at the base of the pulmonary trunk and at the base of the aorta
- septum
- (plural = septa) walls or partitions that divide the heart into chambers
- sulcus
- (plural = sulci) fat-filled groove visible on the surface of the heart; coronary vessels are also located in these areas
- superior vena cava
- large systemic vein that returns blood to the heart from the superior portion of the body
- systemic circuit
- blood flow to and from virtually all of the tissues of the body
- trabeculae carneae
- ridges of muscle covered by endocardium located in the ventricles
- tricuspid valve
- term used most often in clinical settings for the right atrioventricular valve
- valve
- in the cardiovascular system, a specialized structure located within the heart or vessels that ensures one-way flow of blood
- ventricle
- one of the primary pumping chambers of the heart located in the lower portion of the heart; the left ventricle is the major pumping chamber on the lower left side of the heart that ejects blood into the systemic circuit via the aorta and receives blood from the left atrium; the right ventricle is the major pumping chamber on the lower right side of the heart that ejects blood into the pulmonary circuit via the pulmonary trunk and receives blood from the right atrium
Contributors and Attributions
OpenStax Anatomy & Physiology (CC BY 4.0). Access for free at https://openstax.org/books/anatomy-and-physiology