Life-stage dependent response of the epiphytic lichen Lobaria pulmonaria to climate
- 1Department of Biology, University of Florence, Florence, Italy
- 2Department of Earth Sciences, Environment and Life (DISTAV), University of Genoa, Genoa, Italy
- 3Department of Pharmacy (DIFAR), University of Genoa, Genoa, Italy
- 4Department of Biological, Geological and Environmental Sciences, University of Bologna, Bologna, Italy
- 5Department of Biology, Ecological Modeling, University of Hamburg, Hamburg, Germany
Lichens are poikilohydric organisms, whose internal water content tends to reflect external humidity conditions. After drying, they can reactivate their metabolic activity through water vapor uptake or liquid water input. Thus, lichen water-related functional traits are important as they are involved in the duration of the hydrated period. Models predicting the effect of environmental conditions on lichens are based mainly on the presence or absence of adult thalli. Nevertheless, ecological conditions required by lichens might vary during their life cycle, for example during propagule establishment or in the first stages of thallus development. Little is known about the different ecological requirements at the different development stages in lichens. In this work, we measured water holding capacity (WHC) and specific thallus mass (STM) of adult and juvenile thalli of the model species Lobaria pulmonaria along a climatic gradient to constrain the process-based model LiBry. The LiBry model allows accounting for the productivity of lichens with different physiological strategies under various environmental conditions. We simulated the activity and performance of adult and juvenile thalli in 9 regions of Italy and Corsica. The model was used to test if adult thalli of L. pulmonaria have a higher survival probability due to their higher aerodynamic resistance. In the current climatic condition, the LiBry model predicts a higher survival probability of adults with decreasing absolute survival rates of both life stages with increasing temperature. Adult thalli also result in having higher active time, STM, and relative growth rate (RGR). We discuss the main implications of our simulation outputs, provide future perspectives and possible implementations of the LiBry model.
Introduction
Human-induced atmospheric CO2 rising has led to temperature increase together with more frequent and intense drought events (Astigarraga et al., 2020). Numerous negative effects have already been reported to potentially affect biodiversity, for instance species distributional shift, phenological changes, and variation in population dynamics (Bellard et al., 2012). However, not all organisms are affected in the same way: different ecological and physiological characteristics determine their sensitivity to warming (Pörtner and Farrell, 2008; Paaijmans et al., 2013). Lichens, for example, being poikilohydric organisms, are not able to regulate their water content, which tends to reflect external conditions (Proctor and Tuba, 2002). Consequently, both water availability and temperature are fundamental in determining the duration of their hydrated periods, influencing their physiology and, as a consequence, their growth (Gauslaa, 2014). In general, lichen ecophysiological performance depends on external environmental conditions that regulate their photosynthetic and respiratory activities (Green and Lange, 1995; Green et al., 2008). This strong dependence on external climatic factors makes them susceptible to climate change (Nash and Olafsen, 1995). This effect is exacerbated in the Mediterranean region, where a reduction of precipitation and warming are expected (Giorgi and Lionello, 2008), thus determining a climate change hotspot (Tuel and Eltahir, 2020).
Lichens cannot actively control water loss but they can recover their metabolic activity at low water potentials, through water vapor uptake or liquid water input via dew and fog (Gauslaa, 2014). In this framework, identification of key water-related traits in lichens could help determine their susceptibility to environmental factors mainly related to water uptake and loss (Phinney et al., 2018; Phinney, 2019). Water-related traits are involved in the duration of hydrated periods and mediate the response to different sources of water (Gauslaa and Coxson, 2011; Gauslaa, 2014; Di Nuzzo et al., 2022a). A high intraspecific variability has been observed in such traits, reflecting their fundamental role in acclimation to local conditions of water availability (Merinero et al., 2014; Longinotti et al., 2017; Wan and Ellis, 2020). Increasing specific thallus mass (STM), for example, which strongly drives water holding capacity (WHC), has been observed to increase in dry and high light conditions or in the drier part of the year (Larsson et al., 2012). Intraspecific variability in STM and WHC is also associated with thallus size and age (Merinero et al., 2014), leading to different ecological requirements at different life stages as observed, for example, for Lobaria pulmonaria Hoffm (Benesperi et al., 2018; Ignatenko et al., 2020). The model species L. pulmonaria is a foliose green-algal lichen (Figure 1) with cyanobacteria in small internal cephalodia. It is mainly restricted to humid old-growth forests characterized by ecological continuity, and it is often associated with other rare species. During the last centuries, its presence has declined due to forest management and air pollution (Scheidegger and Werth, 2009; Paoli et al., 2019). This negative trend is expected to be worsened by both direct and indirect effects of climate change. Directly, by a reduction of its climatic suitability in the geographical space (Nascimbene et al., 2016) and indirectly through climatic-induced loss of suitable substrates, such as host trees (Nascimbene et al., 2020). Thus, different environmental filters will shape the adult population, to such an extent that even the fine-scale location of the juvenile thalli may determine their survival and development success (Benesperi et al., 2018). It is, therefore, crucial to consider population structure and development stages when testing the effect of climate change on lichens (Benesperi et al., 2018; Bianchi et al., 2020; Di Nuzzo et al., 2022b).
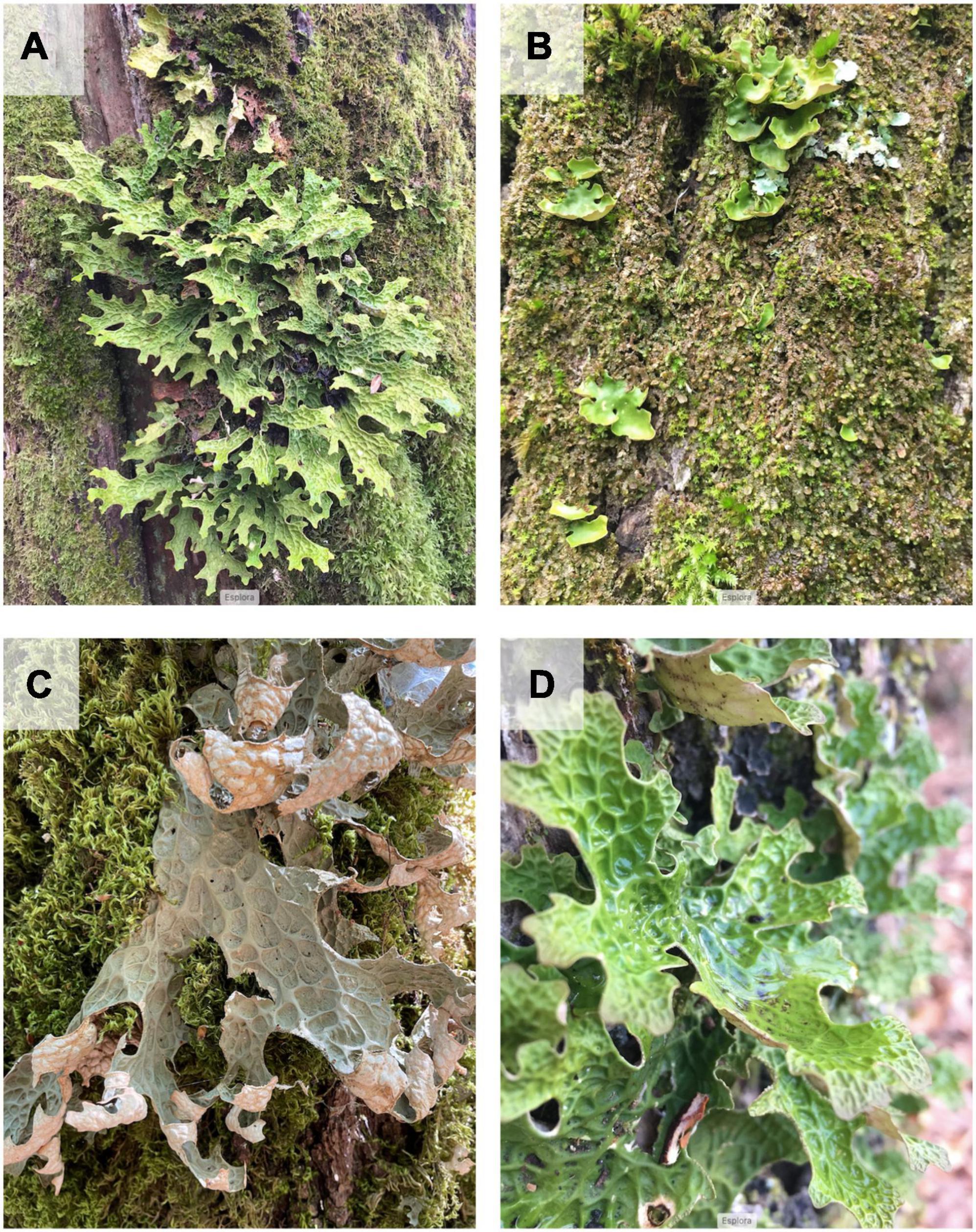
Figure 1. Life stages of Lobaria pulmonaria as considered in this study: (A) adult thallus with downward growing lobes that tend to overlap leaving an air space between the different levels; (B) juvenile thalli at the beginning of development. The differentiation between ascending and descending lobes is still in the early stages, but the lobes are not yet overlapping; (C) adult thallus in the dehydrated state with convoluted apex of the lobes; (D) detail of an adult thallus in the hydrated state in a natural situation after heavy rain. The accumulation of external water favored by the spoon-shaped conformation of the lobes is visible.
Predicting species responses to climate change can be a challenging task, yet it is fundamental to provide effective management policies to counteract the negative effects of rising temperature (Bellard et al., 2012). Correlative models use the currently recognize niche of a species, comparing it with future climatic conditions (Pacifici et al., 2015). By contrast, mechanistic simulation models can be valuable complementary tools because they allow projections to be made in time and space by analyzing underlying physiological processes that lead to the observed effects of climate change on organisms (Pacifici et al., 2015). Mechanistic modeling through detailed small-scale information offers the possibility to define niche estimates to reflect the specific niche characteristics of locally adapted individuals (Peterson et al., 2015). Moreover, this approach allows for accounting for both population structure and possible differences in ecological requirements at different life stages. So far, this has mostly been done for vascular vegetation, such as forest stands, for instance (Fisher et al., 2018). As mechanistic models are often more time-consuming in their development and require a large amount of observational data for evaluation, in ecology, they are less used than correlative models. One of the advantages that we want to highlight of a mechanistic model, is the possibility of switching processes on and off, and examining them separately, to gain an understanding of their role and relevance. Such a modeling approach indeed requires making basic assumptions that facilitate the link between environmental conditions, available resources, and ecological interactions (Pontarp et al., 2019).
Regarding lichens, previous studies mainly modeled ecological niches and patterns taking into account adult thalli (Nascimbene et al., 2020; Ellis and Eaton, 2021; Vallese et al., 2021). Thus, less is known on the effects of climatic conditions on different life stages in lichens. Moreover, different ecological requirements at different life stages are fundamental to determining the survival of a population. Some steps of the life cycle are more affected by climatic conditions and are extremely critical for population development, for instance, dispersion and establishment of propagules. In fact, some traits of the organisms could change during development. For example, the WHC could be different at different life cycle points determining a non-homogeneous probability of occurrence of a thallus along the whole life cycle.
This study aims at testing the hypothesis that adult Lobaria pulmonaria thalli better survive under given climatic conditions than juveniles, due to slower water loss rate related to higher aerodynamic resistance. This advantage may overcompensate the benefit of a low STM in juveniles, which means that juveniles may require less carbon assimilation to achieve the same given relative growth rate (RGR).
We tested this hypothesis using the process-based non-vascular vegetation model LiBry (Porada et al., 2013). The LiBry model accounts for the productivity of lichens in many physiological strategies under a broad range of environmental conditions (Porada et al., 2013).
Materials and methods
Study area and sampling design
For the purpose of this work, we have established some operational definitions, which are described below:
– Region: each of the 9 ERA5 grid cells located in different areas of Italy and selected for this study. Each region contains a number of sites ranging from 1 to 14, depending on the local abundance of L. pulmonaria.
– Sites: areas within the regions where biological populations of L. pulmonaria are present.
– Plot: each of the 30 × 30 m areas located within each site and used to statistically select samples of L. pulmonaria.
– Population: the biological population of L. pulmonaria present at each of the selected sites.
Sites were selected randomly among all localities known to host L. pulmonaria in Italy and Corsica (Nascimbene et al., 2016). The selection was done trying to represent all different ecological conditions (climate, substrata, habitat) in which L. pulmonaria is present in Mediterranean environments. We selected 50 sites in 9 regions across Italy and Corsica (Figure 2). In accordance with Benesperi et al. (2018), we selected 2 life stages of the thalli: (i) juvenile thalli (with an area ≤ 1 cm2, lacking sexual or vegetative diaspores) and (ii) adult thalli (with sexual or vegetative diaspores). In each site, we randomly selected a 30 × 30 m plot in which at least 3 trees with a minimum of 10 adult thalli of L. pulmonaria occurred. In each plot, the number of adult thalli was counted. We considered a thallus an individual if it was completely separated from the closest thallus (> 2 cm). We then randomly collected 5–15 lobes, proportionally to the overall number of adult thalli. To avoid damage to L. pulmonaria populations, we collected only the last 5 cm of each lobe. In order to have a variable range of measurements without harming the population, we also collected 4–5 samples of juvenile thalli of L. pulmonaria in a subset of populations where it was possible (i.e., where the abundance of juveniles was higher enough so that the collection would not cause a threat to the population).
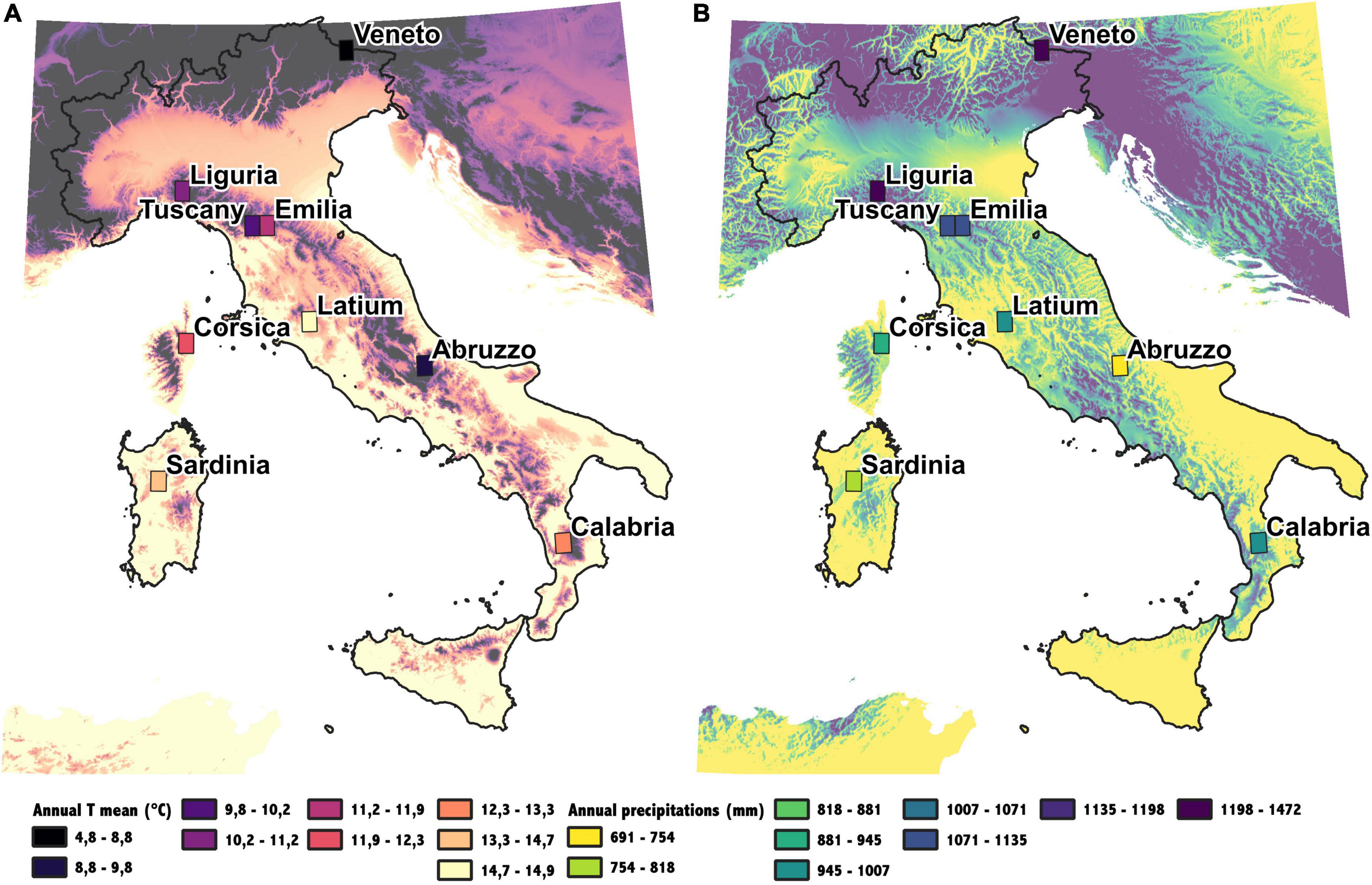
Figure 2. Climatic conditions in the studied area. Annual mean temperature (A) and Annual precipitation (B). The rectangles indicate ERA5 pixels used for each site, the color inside rectangles is the mean value of temperature and precipitation.
Water-related traits
The measurement of water-related traits was performed according to Longinotti et al. (2017). The upper surface of collected thalli was sprayed with deionized water reaching full hydration, i.e., continuing spraying would not induce wet mass gain. The water mass (WM) was then measured in three subsequent steps. Thalli were measured when (i) fully water-saturated (WMmax), (ii) after being gently shaken until no more drops were falling (WMshaking), and after being blotted with dry filter paper (WMblotted). The hydrated thalli were then scanned with a Canon i-SENSYS MF4320d (Canon Inc., Tokyo, Japan). Images obtained from scansion were processed digitizing the thallus outline using Photoshop CS6 Extended (Adobe Systems, San Jose, CA, USA) to measure the projected area (Awet). Thalli were dried at environmental conditions and weighted to measure dry mass (DM).
STM was calculated as DM/Awet. In order to calculate WHCmax, WHCshaking and WHCblotting we used respectively, WMmax, WMshaking and WMblotting as WM in the equation (WM-DM)/Awet. Finally, we calculated the external WHC (WHCext) as WHCshaking -WHCblotting, while WHC internal (WHCint) was assumed to be equal to WHCblotting. Moreover, we calculated percent water content (WC) as (WM-DM)/DM both after shaking and blotting.
Thallus thickness was estimated by 3 measures on a thin section of the thallus under a standard light microscope.
For a subset of lobes, we measured the WMblotting loss halftime (T50). All lobes were hydrated for 24 h in distilled water. Lobes were then left dehydrated at air temperature and humidity and weighted with a time interval of 5 min on an analytical scale until the desiccation at room temperature was reached. The evaporation process could be described as,
Where kwl is water loss rate t1/2 and water loss halftime. Samples kwl and t1/2 were calculated following the exponential function which better approximates the experimental points. The dehydration curve was constructed interpolating 10 subsequent weights.
The model: LiBry
To test our hypothesis, we apply here a mechanistic computer simulation model of lichens, bryophytes, terrestrial algae, and cyanobacteria, called LiBry. This model was developed as a dynamic global vegetation model (DGVM) which, in contrast to most other DGVMs, focuses on non-vascular vegetation, while trees, shrubs, and grasses are only included as a relatively static environment which influences the growth of non-vascular organisms (Porada et al., 2013). The original purpose of the LiBry model was to assess the impact of non-vascular vegetation on global biogeochemical cycles, such as cycles of carbon, water, and nitrogen, and it has been applied to various research questions in this regard (Porada et al., 2013, 2014, 2018, 2019).
The model, however, is not limited to biogeochemical processes alone, it also accounts for factors that shape the physiological diversity of non-vascular communities. Hence, impacts of environmental factors, such as climate, on community composition or on individual species can be assessed using LiBry (e.g., Porada et al., 2019; Baldauf et al., 2021). This is possible through the explicit representation of the large physiological and morphological diversity of non-vascular communities in the model. To this end, LiBry simulates a large number (thousands) of different strategies at the same time in a given location. Each of these strategies is characterized by a unique combination of values of 11 physiological and morphological traits. This means that real non-vascular species can be described by the model to the level of the phenotype. Thereby, one strategy may match individuals from more than one species in the real world, since these may be highly similar in their trait values. Moreover, individuals from the same species may correspond to different strategies in the model, in case of high intra-specific variation in trait values. The traits which are considered in LiBry include STM, WHC, thallus thickness, optimum temperature of photosynthesis, or photosynthetic capacity.
At the beginning of a LiBry simulation, the ranges of possible trait values, which are based on literature, are randomly sampled to create a set of initial physiological strategies (see Porada et al., 2013 for details). Subsequently, the long-term carbon balance of each strategy under given climatic conditions is computed by the model. Differences in trait values usually result in a divergence of the carbon balance values of the simulated non-vascular community at a given location. Consequently, the carbon balance is used as a selection criterion for the success of each strategy: Those strategies which show a negative carbon balance in the long term are removed from the simulation, and the remaining ones are weighted according to a scheme, which is based on growth and other properties, and which determines their relative abundance and, thus, the community composition at the respective location. It should be noted that WHC values reported here (WHCLiBry) do not correspond to the WHC of a single individual, but represent the community mean WHC weighted by relative abundances of the simulated strategies. Hence, to compare WHCLiBry to observations, the measured samples should be representative of the entire community in a given location.
The biomass of each strategy in the LiBry model corresponds to their accumulated long-term carbon balance. Biomass dynamics depend on Net Primary Production (NPP) and mortality, where the latter is based on tissue turnover and disturbance. Moreover, biomass translates into the relative cover fraction of a strategy in the community, depending on its STM. Thereby, the RGR of the cover of a strategy corresponds to the difference between NPP and tissue turnover. In a steady state of the cover, the RGR is balanced by disturbance. It should be pointed out that RGR is a spatially averaged property that integrates processes such as dispersal and establishment. It cannot be compared to the growth rate of an individual thallus, it is rather the average expansion of a population of thalli which share the same trait values. NPP is computed as the difference between photosynthesis and respiration. The former is estimated as a function of light, CO2, and temperature according to Farquhar and von Caemmerer (1982), and the latter is derived from a Q10-relation to temperature. While these basic processes are similar in vascular and non-vascular organisms, the LiBry model also explicitly accounts for physiological properties which only occur in non-vascular vegetation. These include poikilohydry, which means a lack of active control on water loss, the exchange of water via the thallus surface, due to the absence of roots and stomata, the ability to deactivate metabolism upon desiccation, and the reduction of CO2 diffusion at high water saturation of the thallus. Thereby, simulated metabolic activity of the strategies in LiBry is based on the (dark) respiration rate which increases with water saturation. Usually, full activity is reached before the thallus is saturated with water.
Furthermore, several physiological processes in LiBry are connected via trade-offs, which are characteristic for non-vascular organisms and which have a large influence on carbon balance, and, consequently, success in the simulated selection. These trade-offs include a positive correlation between photosynthetic capacity and specific respiration rate, or the negative correlation between metabolic activity and CO2 diffusivity, mediated by water content (see Porada et al., 2013; and Porada and Giordani, 2021 for a more detailed description).
Adaptation of LiBry to Lobaria pulmonaria
To represent both adult and juvenile thalli of L. pulmonaria in the LiBry model, the representation of (a) water pools in the lichen thallus and (b) the morphology of the thallus was extended for this study, compared to the latest published LiBry version (Porada and Giordani, 2021). Furthermore, the initial strategies in LiBry were constrained by observational data.
In LiBry, water in any part of the thallus can be stored in two pools: (1) inside the cells (internal), which expand upon water uptake, or (2) between the cells (external), either in the pore space or attached to the surface of the thallus (see Figure 3). The remainder of the thallus part is filled with air. Under natural conditions, a certain fraction of the thallus (free pore space) always remains air-filled due to either hydrophobicity or pore size effects. In lichens the free pore space usually corresponds to parts of the medulla. Since LiBry simulates a large variety of different physiological strategies, the relative fractions of dry matter of the cells, storage capacities for internal and external water, and free pore space vary largely between the strategies with which the model is initialized. Thereby, however, a fixed relation between the amount of cell dry matter and the amount of internal water is assumed, which means that the cells are limited in their capacity to expand. This is supported by various studies on the relation of STM to WHCint (e.g., Gauslaa and Arsenault, 2020) (see Figure 4). Furthermore, in the LiBry version that is applied here, the transition point to full metabolic activity corresponds to the saturation of the internal water pool. Once all cells are fully turgid, activity is assumed to remain constant.
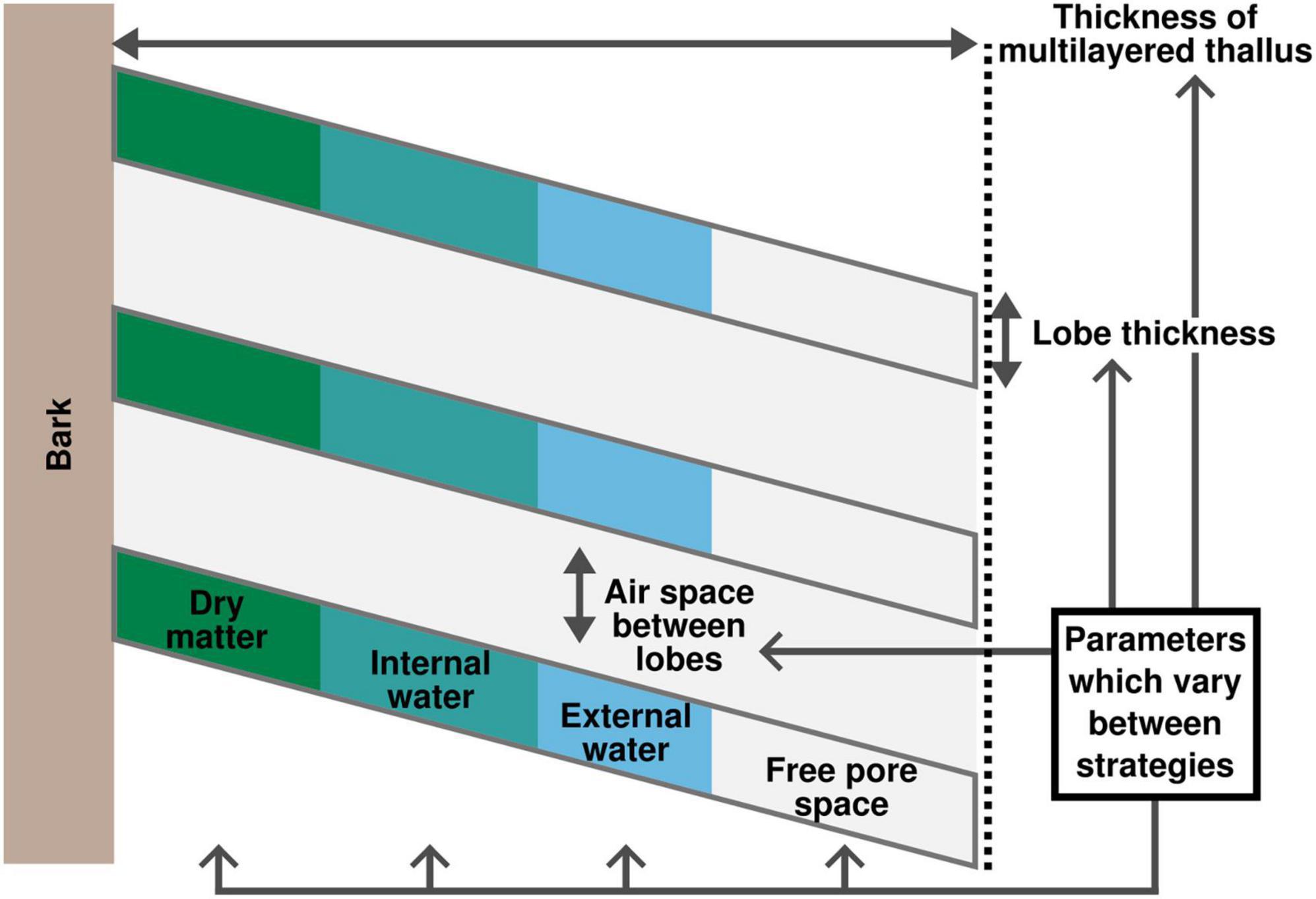
Figure 3. Overview on the morphological representation of L. pulmonaria: in the LiBry model. The individual morphological properties (fractions of dry matter, internal/external water, air in the free pore space inside lobes and air between lobes, lobe thickness, and total thallus height) vary between the strategies in the model. The ratio of dry matter to internal water, however, is constant across strategies.
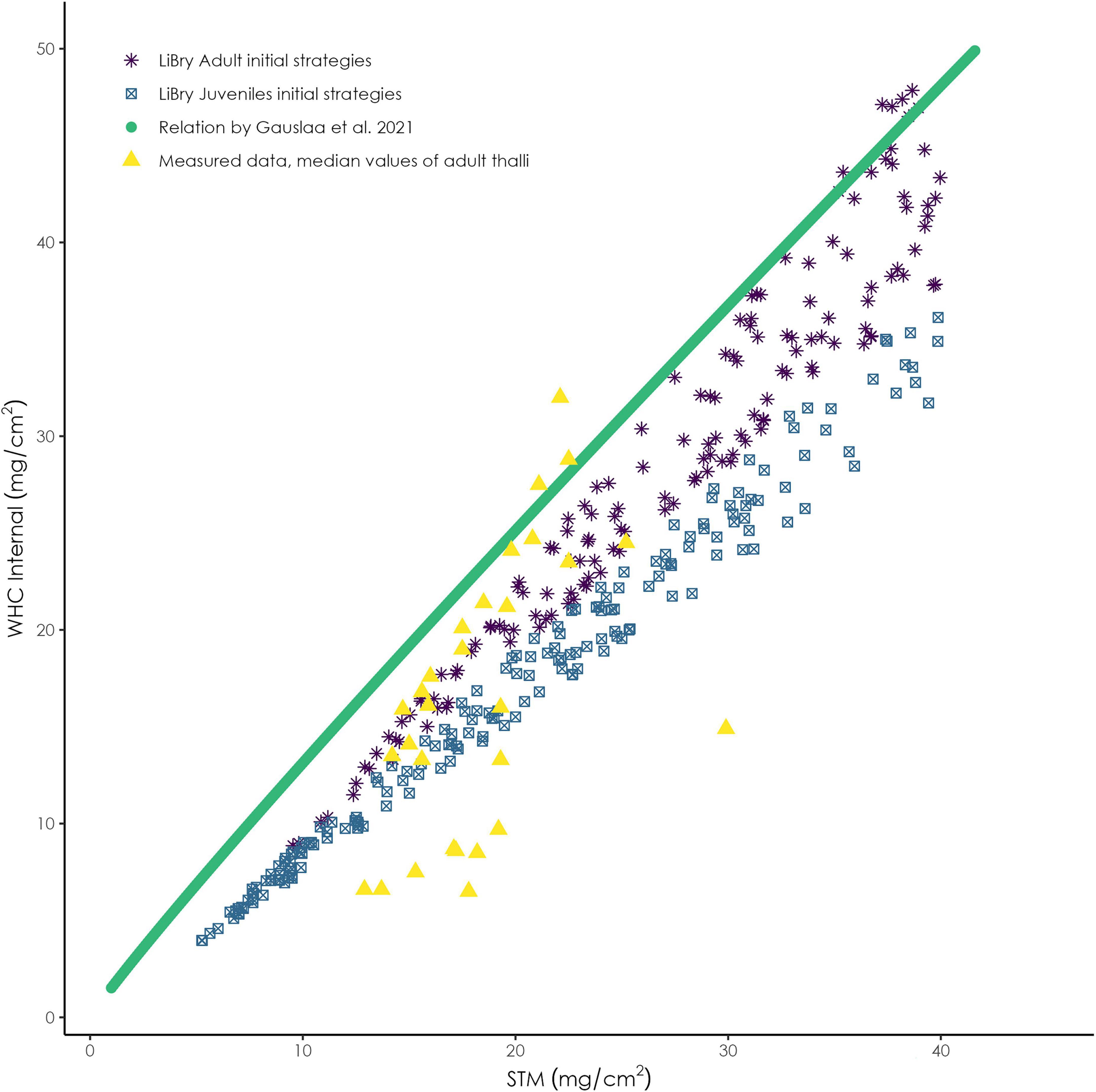
Figure 4. Relation between STM and internal WHC for the initial adult and juvenile strategies in the LiBry model and of measured adult thalli. The scatter in the strategies results from the free variation of several morphological parameters in the model, such as thallus height and the relative fractions of water and air in different parts of the thallus.
To account for the characteristic lobe structure of L. pulmonaria, an additional scaling relation was introduced in LiBry, which prescribes a minimum of air space between lobes, and which leads to relatively more air space in multilayered thalli with a higher thickness. Furthermore, thicker thalli are slightly more efficient in storing water in this model version due to the more complex surface structure (Figure 3).
Several further constraints were applied to LiBry in order to represent L. pulmonaria: First, the value of the fixed ratio between cell dry matter and internal water was set similar to that found for L. pulmonaria by Gauslaa et al. (2021). It was, however, adapted subsequently to observations from the sites used in this study (see Figure 2 and Table 1). Thereby, adult thalli have a higher fraction of WHCint compared to juvenile thalli for the same STM. This is due to the prescribed lower efficiency of water storage per STM for smaller thalli in the model, which was motivated by the observed lower ratio of internal to WHCext in juvenile thalli (Table 1). In this simplified scheme, it is possible that juvenile thalli have STM values similar to adult thalli in the LiBry model, since a small strategy (low overall thickness) may have a high fraction of dry matter, and a tall strategy a substantially lower dry matter fraction. While it is likely that juvenile thalli have a lower STM on average than adult ones, the low number of measurements of STM of juveniles does not allow for a definite distinction between juveniles and adults in this regard. To test the implications of this simplification, we run a sensitivity analysis (see below).
Secondly, the thickness of the lobes (Figure 3) was constrained to the range of 200–1,000 μm, based on observations from the sites (Table 1), and the maximum thickness of the total multilayered thallus, meaning the distance between the bark surface and the outer tips of the lobes, was set to 10 cm, which means that all strategies differed in their thickness, but could not exceed 10 cm. Finally, initial strategies which had an STM outside the range of 4–40 mg/cm2 were excluded from the model, and those strategies which had a ratio of external/internal WHC outside the range of 0.21–2.7 were excluded, too. These ranges were based on Median values of observations from the sites, using half the minimum and twice the maximum value, except for max. STM, which would have increased the average STM of the initial strategies too strongly.
According to our hypothesis (see above), the taller structure of adult thalli may lead to an increased resistance against evaporation of water compared to juvenile thalli. This was tested and confirmed in the laboratory using a subset of sampled L. pulmonaria thalli. Subsequently, we used the evaporation scheme built into the LiBry model to reproduce the observed drying dynamics. For the adult and juvenile thalli, we set WHC to 14 and 7.6 mg/cm2, respectively, and the observed T50 time was 24 min for adults and 12 min for juvenile thalli (median values). Environmental conditions for the evaporation model were set to those of the laboratory (20°C and 60% RH, and the surface resistance of the thalli was used as the calibration parameter to match the observed difference in T50 time.
It was found that the difference in WHC alone could not explain the longer T50 time of adult thalli. Instead, it was required to set the resistance of the juvenile thalli to 0.3 times that of the adult thalli, all other conditions being equal, to match the observations. Therefore, we then used the thallus height Z of a strategy in the LiBry model to define its surface resistance, prescribing an exponential scaling from the minimum height zMIN of 5 mm to the maximum zMAX of 10 cm:
with rS_MAX = 200 s/m. This simple relation resulted in median values of the juvenile initial strategies which were roughly 0.3 times that of the adult initial strategies.
Simulation setup
To differentiate between adult and juvenile individuals of L. pulmonaria strategies in the LiBry model, we used only the thickness of the multilayered thallus. Individuals which were smaller than 1 cm were assigned to the juvenile group, and the remainder to the adult group. Figure 5 shows the distributions of STM, WHCint, and the WHCext/int ratio for the initial strategies in the LiBry model, and also for the observational data from Table 1.
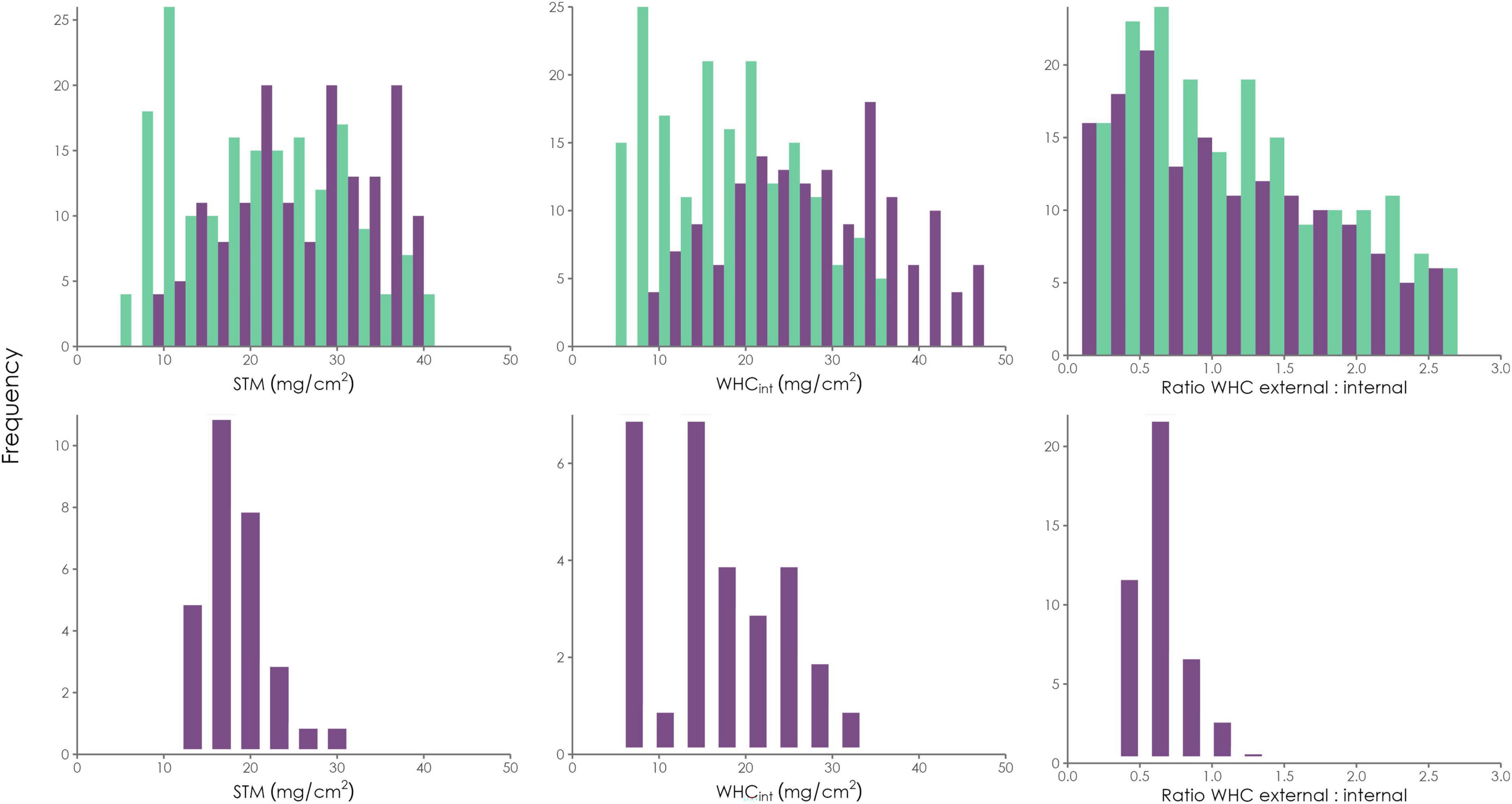
Figure 5. The initial LiBry strategies are separated into juvenile thalli (light green) and adult ones (dark purple). Frequency distributions of STM, internal WHC, and the ratio of external to internal WHC, both of the initial strategies in the LiBry model (Upper row), and also of the observed adult thalli (Lower row) of which only median values are shown.
Two simulations were run with the LiBry model, which only differed with regard to the group of initial strategies (juvenile vs. adult). The simulations were carried out for 9 regions across Italy (Figure 2) and the model was driven by climate data (years 1979–2019) from the ERA5 data set (Hersbach et al., 2020). Those grid cells were cut out from the global data set which corresponds roughly to the center of the individual sites in the 9 respective regions.
Leaf area index was derived from site observations, by imposing a seasonal variation (monthly resolution) on median LAI values for each region. The extent of the variation was based on a previous study carried out in Sardinia (Porada and Giordani, 2021). Stem area index was set to a constant value of 0.1, also based on the study by Porada and Giordani (2021). Note that the SAI in the LiBry model is used to obtain correct estimates for ecosystem level extensive properties, such as biomass or productivity, since per-area growth needs to be scaled up spatially. Since we focus in this study on differences in the energy and water balance between juvenile and adult individuals of L. pulmonaria, the exact area which is available for growth on the stems is not of crucial relevance here.
The simulations were run using 3,000 initial strategies, out of which 632 remained after constraining their properties to characteristics of L. pulmonaria. The juvenile and adult groups consisted of 359 and 273 strategies, respectively. The simulation was run for 300 years to ensure equilibrium with regard to community composition, thereby repeating the 41-year climate data. At the end of each simulation, the number of surviving strategies and their community-averaged properties were analyzed. Thereby, all strategies were assigned equal weights in the averaging. Finally, a sensitivity analysis was carried out to test the effects of different uncertain morphological and physiological properties and environmental conditions on the outcome of our study. This run is carried out for one site only with the same setup as used in a preceding publication (Porada and Giordani, 2021), but applying the new model version.
We want to point out here that LiBry is a deterministic model, which means that each of the strategies is simulated as an individual, consisting of dynamic pools of carbon and water, which are changing based on the state of the individual and the driving environmental conditions. Hence, for the same set of initial strategies and climate data, the model will always calculate the same output values. When comparing differences between the simulations for adult and juvenile strategies, it is thus not appropriate to test if these differences are significant, since the model estimates cannot be interpreted as draws from a statistical population. It is rather the generation of the initial strategies itself which corresponds to statistical sampling. Here, it is important to test if the sample size of strategies is large enough to ensure consistent results. This is done in the last part of our sensitivity analysis.
Results
Simulation output
The results of our simulations with the LiBry model confirmed our overall hypothesis. Adult strategies of L. pulmonaria had a 32% higher survival rate than juveniles (comparison of the median values of all regions, see also Figure 6). Adult strategies show a higher fraction of active time compared to juveniles (Act.Frac.). They also have a higher (WHC_LiBry), and a higher RGR, see also Table 2.
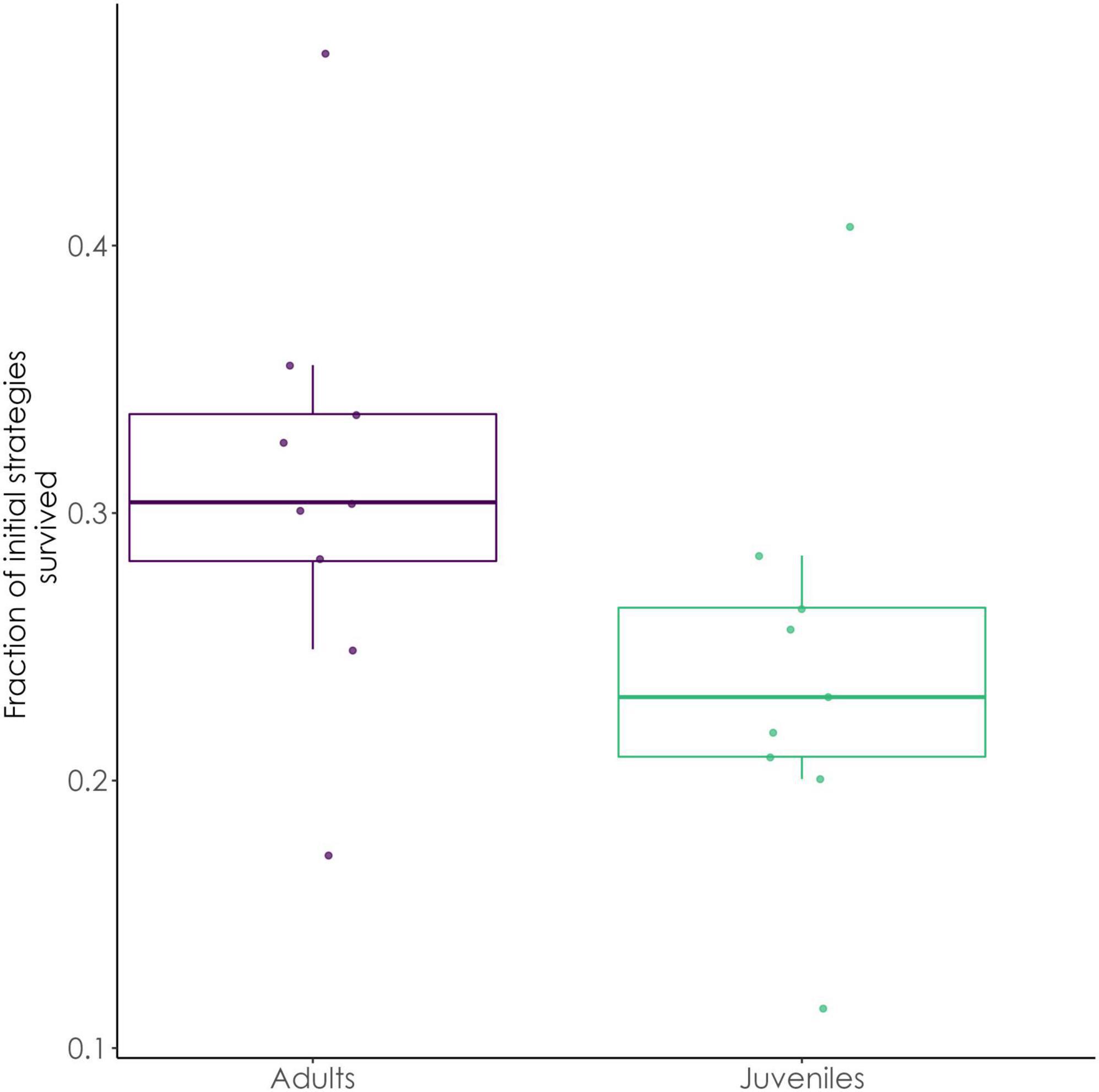
Figure 6. Boxplots of the fraction of initial strategies which survived the simulation, adult (Left) vs. juvenile thalli (Right). The boxplots contain 9 values each, corresponding to the different regions of Italy analyzed here. Colored bars represent the density of values.
When the 9 regions are sorted along climatic gradients, certain patterns in the ratios of survival rates and physiological functioning of adult vs. juvenile strategies can be noted (Figure 7 top panels). Increasing total amount of rainfall (range: 691–1,472 mm/a) does not seem to result in a clear pattern in the ratios of survival rate or the physiological functions (WHC, Act.Frac., RGR), and also absolute survival rates of adults and juveniles show no clear trend (Figure 7 middle panels). Instead, survival rates seem to be lowest at intermediate amounts of rainfall. Increasing mean air temperature (range: 4.8–14.9°C), however, seems to lead to an increase in the relative survival rate of the adult strategies compared to juvenile ones, and to a decrease in the difference in WHC. RGR seems to be connected to survival rate, while active time fraction does not show a clear pattern or a strong correlation to other ratios. For high air temperature, absolute survival rates show a decrease for both adult and juvenile thalli. Moreover, it should be pointed out that the ratio of adult to juvenile survival is always larger than one, which means that the simulated advantage of adult thalli is consistent throughout the study region.
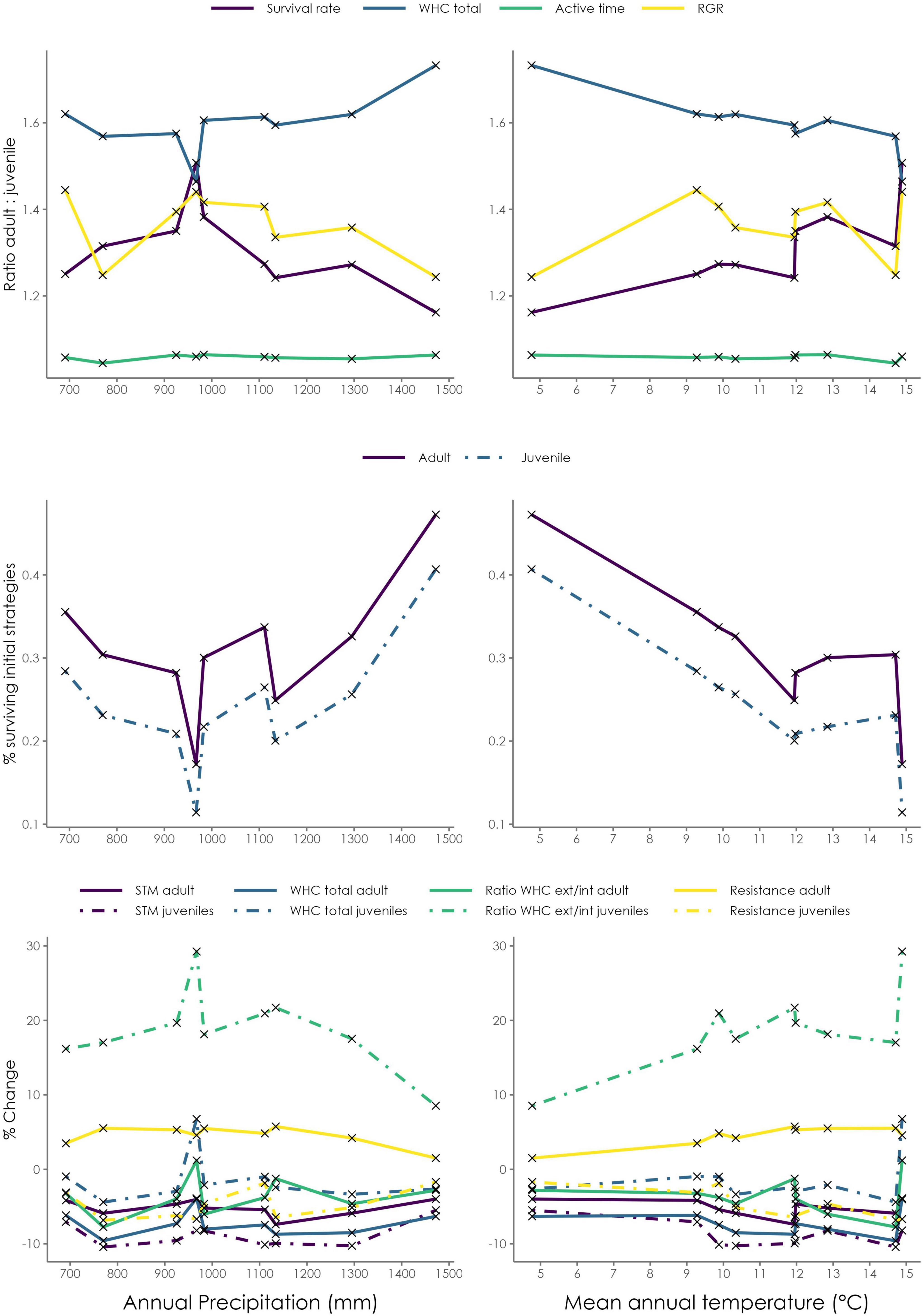
Figure 7. (Top) Simulated ratios of adult to juvenile survival rate (purple), Water holding capacity—WHCLiBry (blue), Active fraction—Act.Frac. (yellow) and Relative Growth Rate—RGR (yellow) for the 9 regions in Italy examined here. The climate variable increases from left to right, total rainfall (Left), and average air temperature (Right). (Middle) survival rates of adult (purple) and juvenile (blue) thalli. (Bottom) Shift in the community mean values of four hydration traits from the initial strategies to those surviving at the end of the simulation; STM (purple), WHCLiBry (blue), the ratio of external to internal WHC (green), and resistance against evaporation (yellow) are shown separately for adult thalli (solid) and juvenile ones (dashed).
When considering adult and juvenile thalli separately, and comparing the shift in their mean properties (due to the selection in the LiBry model), it becomes clear that the ratio of external to internal WHC in juveniles exhibits the strongest shift from initial to final mean properties (Figure 7 bottom panels). This means that juvenile strategies are selected for higher external: internal WHC ratio during the simulation, but not adult thalli. Another consistent pattern is the selection for slightly higher resistance against evaporation in adult thalli, but not in juveniles. Other properties, such as STM and WHCLiBry, show a slight decrease in the mean values, but no clear pattern along the climatic gradients. Another feature seems to be the increase in WHCLiBry for juveniles in regions with the lowest survival rates.
Sensitivity analysis
The sensitivity analysis confirmed our assumptions about the direction of the impacts of physiological and environmental properties on the survival of strategies in the LiBry model:
(1) When the physiological disadvantage of juvenile strategies due to the reduced resistance against evaporation is switched off in the model, and the maximum resistance value rS_MAX is used for every strategy, the survival rates increase overall and the adult strategies have only a 13% higher survival rate than the juvenile ones. This suggests that more than half of the advantage in the survival of adult vs. juvenile thalli depends on their higher resistance against water loss, and the remainder may be due to their higher WHC per area.
(2) It has been observed that lichens show a burst of respiration upon rewetting from the dry state, with magnitude varying between individuals and species (Smith and Molesworth, 1973). In the standard version of LiBry, this resaturation respiration is not included, due to a lack of data on potential trade-offs which may explain the variation in the magnitude of respiration. Hence, we here test the effect of a simple parameterization of resaturation respiration, where a 50% increase in respiration is assumed for 2 h after each rewetting event. As expected, overall survival rates declined compared to the control run. Moreover, the advantage of the adult strategies increases and they show 54% more survival than the juvenile ones.
(3) It has been shown that the bark may act as an important water reservoir for epiphytic lichens (Porada and Giordani, 2021), and utilizing a bark water reservoir in the LiBry model increases survival rates, as expected. The conditions improve to an extent that almost removes the difference between adult and juvenile strategies (6%).
(4) We additionally constrained the dry matter fraction in the model to 5% or less. In this way, juvenile thalli cannot compensate their lower stature (less than 1 cm in the model) by a high fraction of dry matter in the thallus, and thus automatically have a lower STM on average. We thereby account for a potential systematic lower STM of juveniles thalli which is not captured by our simplified model. The modified set of initial strategies does not change substantially the outcome of our simulation (see Tables 3, 4). The WHC of the juveniles is lower, which can be explained by the now prescribed low amount of dry matter that stores water for each strategy. Also, the survival rate of the juveniles is slightly higher now, since those strategies with high STM values were already removed in the initialization of the model, due to the new constraint. In the control version, the average STM of juveniles declined only during the simulation due to selection, leading to fewer survivors compared to the number of initial strategies.
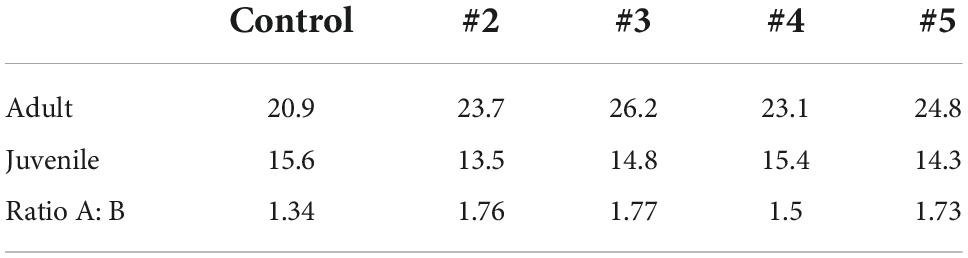
Table 4. Percentage of surviving strategies for 5 different drawings of 3,000 initial strategies from the pool of potential strategies, and the ratio of adult: juvenile survival.
(5) The advantage of adult thalli compared to juvenile ones regarding survival rate is consistent across 5 drawings of 3,000 initial random strategies in the LiBry model, which suggests that this effect is not a random result due to insufficient sample size.
Discussion
The results of our simulations depict for the first time a different survival probability of life stages of L. pulmonaria. The LiBry model highlights a consistently higher probability of adult thalli to survive and more active time. Our simulations set the basis for a better understanding of how climatic gradients affect different age stages of L. pulmonaria, revealing complex population dynamics. L. pulmonaria is known to be influenced by both spatial (Eaton and Ellis, 2012) and seasonal climatic gradients (Larsson et al., 2012). More oceanic climates seem to enhance biomass gain and reduce the age-at-reproduction (Eaton and Ellis, 2014 and references therein). Nevertheless, also microclimatic and microhabitat conditions, nested inside macroclimatic gradients, influence the growth rate and the overall survival of L. pulmonaria populations (Benesperi et al., 2018; Ellis, 2020; Di Nuzzo et al., 2022b). For example, the same distance from watercourses in two different areas could lead to different growth rates (Ellis, 2020).
A consistent outcome of the simulation of L. pulmonaria along climatic gradients is that the advantage of adult thalli compared to juvenile ones, regarding survival, increases with decreasing absolute survival rates of both life stages, which can be interpreted as stressful conditions. It is interesting that adult and juvenile thalli seem to become more similar with respect to WHCLiBry under these conditions (Figure 7 top panels). A more detailed analysis shows that the relative increase in WHCLiBry in juvenile thalli compared to adult ones seems to be accompanied by a selection of juvenile strategies toward higher ratios of external to internal WHC (Figure 7 bottom panels). This increase in external WHC can be interpreted as a means to maintain active time and, thus, growth under stressful conditions, at lower carbon cost facilitated by a lower fraction of dry matter (which is related to internal WHC). Alternatively, the reduced fraction of internal WHC compared to external one may be driven by the advantage of earlier activation, since only internal WHC is relevant for the level of metabolic activity in LiBry. Usually, the increased external WHC would also have a negative effect on growth via the associated decrease in CO2 diffusivity (Lange and Green, 1996; Lange et al., 1999). However, it could be possible that this effect is not relevant in warm and dry climatic conditions which seldom lead to full saturation, or where evaporation rapidly decreases the external water. In contrast to juvenile strategies, adults do not show a shift toward higher WHC, but they instead have slightly increased their resistance against evaporation. A plausible explanation for these contrasting patterns is the limitation of juvenile thalli to a height of 1 cm in the model. Since we parameterized resistance against evaporation as a function of height, juvenile strategies are constrained in their ability to increase surface resistance. The lack of simulated increase in external: internal WHC ratio in adult thalli as an additional means to increase active time may be explained by their larger overall WHC. Due to this, periods of higher water saturation after rainfall may be prolonged compared to juveniles and they may be more often limited by CO2 diffusion under these conditions if water is stored externally. This may also explain why the external: internal ratio only increases at high temperature for adult thalli, since they may be more frequently dry then. Merinero et al. (2014) demonstrated that the preferred source of water between younger and adult thalli shift from air humidity to liquid water. The increment of STM in adult thalli increases the amount of water necessary to achieve full saturation, making them more dependent on liquid water. At the same time, thicker thalli could prolong the hydrated periods prolonging the photosynthetic activity.
Finally, the slight decrease in STM and WHCLiBry for both adult and juvenile strategies may be explained by the general disadvantage of high STM under stressful conditions in the model. This is due to the fact that, for the same amount of lateral expansion, a strategy with a high STM has to assimilate more carbon than one with a low STM. Expansion, however, is crucial for survival in LiBry since it needs to compensate for the loss of cover due to disturbance and turnover. In the standard version of LiBry, thallus height, which is associated with high STM under otherwise equal trait values, is connected to a competitive advantage, and thus results in higher relative cover of the surviving tall strategies in the simulated community (Porada et al., 2019). Here, however, we treated all strategies as equally competitive since we focused on ecophysiological processes, and not on potential interactions between different life stages in the same population. The latter process is poorly known, since data on competition between adults and juveniles on single trees are currently not available. We thus wanted to avoid an overestimation of the advantage of adults based on their growth height in the model.
The simulation outputs obtained from LiBry led to two main implications: (1) Warming due to climate change may be harmful to the survival of juvenile thalli. In fact, harsher conditions could exacerbate the difference in terms of survival between adults and juveniles. Moreover, an overall lower active time could lead to delaying the age-at-reproduction, reducing the dispersal capability of such a population. Such effects of warming on L. pulmonaria may be studied through forcing LiBry by climate change scenarios. (2) A monitoring of the metabolic activity of L. pulmonaria thalli of different ages under field conditions along a climatic gradient, combined with laboratory analysis of their water relationships could be used to evaluate in better detail the findings predicted by the LiBry model. In particular, analysis of mean morphological traits at the community level for different locations along the climate gradient could be used to assess the selection process in LiBry.
The present implementation of LiBry does not account for either intra-and interspecific competition and facilitation processes. Nevertheless, these dynamics could be extremely important over long time periods. In the case of L. pulmonaria, interaction with bryophytes could play a facilitation role at an early stage of development, as bryophytes could provide supplementary water enhancing photosynthetic active periods (Benesperi et al., 2018). This is supported by our sensitivity analysis with regard to the bark water reservoir, which may have a similar effect. A possible further application of the LiBry model could be to test the competition and facilitation dynamics at a community level, by setting different interaction schemes and then comparing them to observed community compositions of both lichens and bryophytes. These interactions are probably mediated by different functional traits. For instance, foliose aerohygrophytic chlorolichens, such as L. pulmonaria (Larsson et al., 2012) could benefit from bryophytes species with a growth form that reduces evaporation of water but at the same time could be overgrown by these latter. Crustose lichen species could be outcompeted by fast-growing bryophyte or other lichen species. As demonstrated for Alpine plant communities, complex interactions of facilitation and competition might support higher biodiversity (Losapio et al., 2021).
Populations of L. pulmonaria have strongly declined during the last century, mainly due to anthropogenic disturbance. Moreover, future climate change is predicted to worsen and reduce suitable conditions (Nascimbene et al., 2016, 2020). The results discussed here strengthen the importance of management practices focused on the preservation of specific conditions aimed at enhancing the buffer potential of forests (De Frenne et al., 2021). The temperature under the forest canopy could be up to 2 degrees lower compared to free-air temperature. At the same time, forest structure also influences the quantity and time of different types of water sources (Gauslaa, 2014; Di Nuzzo et al., 2022a). Also, topography could play an important role in buffering harsher climatic conditions, for example protecting forests close to watercourses could be important for providing more humidity (Ellis, 2020). In harsher conditions, where juveniles seem to have a lower possibility of survival, preserving forests with favorable temperature and water conditions could enhance the development of larger populations of L. pulmonaria.
Data availability statement
The raw data supporting the conclusions of this article will be made available by the authors, without undue reservation.
Author contributions
PP and PG designed the study concept together. PP developed the model code and ran the simulations. LD, GC, PG, RB, JN, EB, and AP provided data for model evaluation. LD, GC, PP, and PG wrote the first draft of the manuscript. All authors contributed to the article and approved the submitted version.
Acknowledgments
We would like to acknowledge colleagues and students that helped to collect samples from remote populations.
Conflict of interest
The authors declare that the research was conducted in the absence of any commercial or financial relationships that could be construed as a potential conflict of interest.
Publisher’s note
All claims expressed in this article are solely those of the authors and do not necessarily represent those of their affiliated organizations, or those of the publisher, the editors and the reviewers. Any product that may be evaluated in this article, or claim that may be made by its manufacturer, is not guaranteed or endorsed by the publisher.
References
Astigarraga, J., Andivia, E., Zavala, M. A., Gazol, A., Cruz-Alonso, V., Vicente-Serrano, S. M., et al. (2020). Evidence of non-stationary relationships between climate and forest responses: Increased sensitivity to climate change in Iberian forests. Glob. Chang. Biol. 26, 5063–5076. doi: 10.1111/gcb.15198
Baldauf, S., Porada, P., Raggio, J., Maestre, F. T., and Tietjen, B. (2021). Relative humidity predominantly determines long-term biocrust-forming lichen cover in drylands under climate change. J. Ecol. 109, 1370–1385. doi: 10.1111/1365-2745.13563
Bellard, C., Bertelsmeier, C., Leadley, P., Thuiller, W., and Courchamp, F. (2012). Impacts of climate change on the future of biodiversity: Biodiversity and climate change. Ecol. Lett. 15, 365–377. doi: 10.1111/j.1461-0248.2011.01736.x
Benesperi, R., Nascimbene, J., Lazzaro, L., Bianchi, E., Tepsich, A., Longinotti, S., et al. (2018). Successful conservation of the endangered forest lichen Lobaria pulmonaria requires knowledge of fine-scale population structure. Fungal Ecol. 33, 65–71. doi: 10.1016/j.funeco.2018.01.006
Bianchi, E., Benesperi, R., Brunialti, G., Di Nuzzo, L., Faèkovcová, Z., Frati, L., et al. (2020). Vitality and growth of the threatened Lichen Lobaria pulmonaria (L.) Hoffm. in response to logging and implications for its conservation in mediterranean oak forests. Forests 11:995. doi: 10.3390/f11090995
De Frenne, P., Lenoir, J., Luoto, M., Scheffers, B. R., Zellweger, F., Aalto, J., et al. (2021). Forest microclimates and climate change: Importance, drivers and future research agenda. Glob. Chang. Biol. 27, 2279–2297. doi: 10.1111/gcb.15569
Di Nuzzo, L., Benesperi, R., Nascimbene, J., Papini, A., Malaspina, P., Incerti, G., et al. (2022a). Little time left. Microrefuges may fail in mitigating the effects of climate change on epiphytic lichens. Sci. Total Environ. 825:153943. doi: 10.1016/j.scitotenv.2022.153943
Di Nuzzo, L., Giordani, P., Benesperi, R., Brunialti, G., Fačkovcová, Z., Frati, L., et al. (2022b). Microclimatic alteration after logging affects the growth of the endangered lichen Lobaria pulmonaria. Plants 11:295. doi: 10.3390/plants11030295
Eaton, S., and Ellis, C. J. (2012). Local experimental growth rates respond to macroclimate for the lichen epiphyte Lobaria pulmonaria. Plant Ecol. Divers. 5, 365–372. doi: 10.1080/17550874.2012.728640
Eaton, S., and Ellis, C. J. (2014). High demographic rates of the model epiphyte Lobaria pulmonaria in an oceanic hazelwood (western Scotland). Fungal Ecol. 11, 60–70. doi: 10.1016/j.funeco.2014.03.007
Ellis, C. J. (2020). Microclimatic refugia in riparian woodland: A climate change adaptation strategy. For. Ecol. Manag. 462:118006. doi: 10.1016/j.foreco.2020.118006
Ellis, C. J., and Eaton, S. (2021). Microclimates hold the key to spatial forest planning under climate change: Cyanolichens in temperate rainforest. Glob. Chang. Biol. 27, 1915–1926. doi: 10.1111/gcb.15514
Farquhar, G. D., and von Caemmerer, S. (1982). “Modelling of Photosynthetic Response to Environmental Conditions,” in Physiological Plant Ecology II: Water Relations and Carbon Assimilation, eds O. L. Lange, P. S. Nobel, C. B. Osmond, and H. Ziegler (Berlin: Springer), 549–587. doi: 10.1007/978-3-642-68150-9_17
Fisher, R. A., Koven, C. D., Anderegg, W. R. L., Christoffersen, B. O., Dietze, M. C., Farrior, C. E., et al. (2018). Vegetation demographics in earth system models: A review of progress and priorities. Glob. Chang. Biol. 24, 35–54. doi: 10.1111/gcb.13910
Gauslaa, Y. (2014). Rain, dew, and humid air as drivers of morphology, function and spatial distribution in epiphytic lichens. Lichenologist 46, 1–16. doi: 10.1017/S0024282913000753
Gauslaa, Y., and Arsenault, A. (2020). The cyanolichens Erioderma pedicellatum and Coccocarpia palmicola need much more than a dewfall to fill their water holding capacity. Flora 269:151648. doi: 10.1016/j.flora.2020.151648
Gauslaa, Y., and Coxson, D. (2011). Interspecific and intraspecific variations in water storage in epiphytic old forest foliose lichens. Botany 89, 787–798. doi: 10.1139/b11-070
Gauslaa, Y., Mikulec, M. M., and Solhaug, K. A. (2021). Short-term growth experiments – A tool for quantifying lichen fitness across different mineral settings. Flora 282:151900. doi: 10.1016/j.flora.2021.151900
Giorgi, F., and Lionello, P. (2008). Climate change projections for the Mediterranean region. Glob. Planet. Chang. 63, 90–104.
Green, T. G. A., and Lange, O. L. (1995). “Photosynthesis in Poikilohydric Plants: A Comparison of Lichens and Bryophytes,” in Ecophysiology of Photosynthesis Springer Study Edition, eds E.-D. Schulze and M. M. Caldwell (Berlin: Springer), 319–341. doi: 10.1007/978-3-642-79354-7_16
Green, T. G. A., Nash, T. H. III, and Lange, O. L. (2008). “Physiological ecology of carbon dioxide exchange,” in Lichen biology, ed. T. H. Nash III (Cambridge, UK: Cambridge University Press), 152–181.
Hersbach, H., Bell, B., Berrisford, P., Hirahara, S., Horányi, A., Muñoz-Sabater, J., et al. (2020). The ERA5 global reanalysis. Q. J. R. Meteorol. Soc. 146, 1999–2049. doi: 10.1002/qj.3803
Ignatenko, R. V., Tarasova, V. N., and Markovskaya, E. F. (2020). Ontogenesis of the Lichen Lobaria pulmonaria (L.) hoffm. in plant communities of the boreal zone. Russ. J. Dev. Biol. 51, 115–124. doi: 10.1134/S1062360420020046
Lange, O. L., and Green, T. G. A. (1996). High thallus water content severely limits photosynthetic carbon gain of central European epilithic lichens under natural conditions. Oecologia 108, 13–20. doi: 10.1007/BF00333209
Lange, O. L., Green, T. G. A., and Reichenberger, H. (1999). The Response of Lichen photosynthesis to External CO2 concentration and its interaction with thallus water-status. J. Plant Physiol. 154, 157–166. doi: 10.1016/S0176-1617(99)80204-1
Larsson, P., Solhaug, K. A., and Gauslaa, Y. (2012). Seasonal partitioning of growth into biomass and area expansion in a cephalolichen and a cyanolichen of the old forest genus Lobaria. New Phytol. 194, 991–1000. doi: 10.1111/j.1469-8137.2012.04130.x
Longinotti, S., Solhaug, K. A., and Gauslaa, Y. (2017). Hydration traits in cephalolichen members of the epiphytic old forest genus Lobaria (s. lat.). Lichenologist 49, 493–506. doi: 10.1017/S0024282917000445
Losapio, G., Schöb, C., Staniczenko, P. P. A., Carrara, F., Palamara, G. M., De Moraes, C. M., et al. (2021). Network motifs involving both competition and facilitation predict biodiversity in alpine plant communities. Proc. Natl. Acad. Sci. U.S.A. 118:e2005759118. doi: 10.1073/pnas.2005759118
Merinero, S., Hilmo, O., and Gauslaa, Y. (2014). Size is a main driver for hydration traits in cyano- and cephalolichens of boreal rainforest canopies. Fungal Ecol. 7, 59–66. doi: 10.1016/j.funeco.2013.12.001
Nascimbene, J., Benesperi, R., Casazza, G., Chiarucci, A., and Giordani, P. (2020). Range shifts of native and invasive trees exacerbate the impact of climate change on epiphyte distribution: The case of lung lichen and black locust in Italy. Sci. Total Environ. 735:139537. doi: 10.1016/j.scitotenv.2020.139537
Nascimbene, J., Casazza, G., Benesperi, R., Catalano, I., Cataldo, D., Grillo, M., et al. (2016). Climate change fosters the decline of epiphytic Lobaria species in Italy. Biol. Conserv. 201, 377–384. doi: 10.1016/j.biocon.2016.08.003
Nash, T. H., and Olafsen, A. G. (1995). Climate change and the ecophysiological response of arctic lichens. Lichenologist 27, 559–565. doi: 10.1016/S0024-2829(95)80014-X
Paaijmans, K. P., Heinig, R. L., Seliga, R. A., Blanford, J. I., Blanford, S., Murdock, C. C., et al. (2013). Temperature variation makes ectotherms more sensitive to climate change. Glob. Chang. Biol. 19, 2373–2380. doi: 10.1111/gcb.12240
Pacifici, M., Foden, W. B., Visconti, P., Watson, J. E. M., Butchart, S. H. M., Kovacs, K. M., et al. (2015). Assessing species vulnerability to climate change. Nat. Clim. Chang. 5, 215–224. doi: 10.1038/nclimate2448
Paoli, L., Benesperi, R., Faèkovcová, Z., Nascimbene, J., Ravera, S., Marchetti, M., et al. (2019). Impact of forest management on threatened epiphytic macrolichens: Evidence from a Mediterranean mixed oak forest (Italy). IForest Biogeosci. For. 12:383. doi: 10.3832/ifor2951-012
Peterson, A. T., Papeş, M., and Soberón, J. (2015). Mechanistic and correlative models of ecological niches. Eur. J. Ecol. 1, 28–38. doi: 10.1515/eje-2015-0014
Phinney, N. H. (2019). 3D modelling of thallus topography of Lobaria pulmonaria facilitates understanding of water storage pools. Lichenologist 51, 89–95. doi: 10.1017/S0024282918000531
Phinney, N. H., Solhaug, K. A., and Gauslaa, Y. (2018). Rapid resurrection of chlorolichens in humid air: Specific thallus mass drives rehydration and reactivation kinetics. Environ. Exp. Bot. 148, 184–191. doi: 10.1016/j.envexpbot.2018.01.009
Pontarp, M., Bunnefeld, L., Cabral, J. S., Etienne, R. S., Fritz, S. A., Gillespie, R., et al. (2019). The latitudinal diversity gradient: Novel understanding through mechanistic eco-evolutionary models. Trends Ecol. Evol. 34, 211–223. doi: 10.1016/j.tree.2018.11.009
Porada, P., and Giordani, P. (2021). Bark water storage plays key role for growth of mediterranean epiphytic lichens. Front. For. Glob. Chang. 4:668682. doi: 10.3389/ffgc.2021.668682
Porada, P., Tamm, A., Raggio, J., Cheng, Y., Kleidon, A., Pöschl, U., et al. (2019). Global NO and HONO emissions of biological soil crusts estimated by a process-based non-vascular vegetation model. Biogeosciences 16, 2003–2031. doi: 10.5194/bg-16-2003-2019
Porada, P., Van Stan, J. T., and Kleidon, A. (2018). Significant contribution of non-vascular vegetation to global rainfall interception. Nat. Geosci. 11, 563–567. doi: 10.1038/s41561-018-0176-7
Porada, P., Weber, B., Elbert, W., Pöschl, U., and Kleidon, A. (2013). Estimating global carbon uptake by lichens and bryophytes with a process-based model. Biogeosciences 10, 6989–7033. doi: 10.5194/bg-10-6989-2013
Porada, P., Weber, B., Elbert, W., Pöschl, U., and Kleidon, A. (2014). Estimating impacts of lichens and bryophytes on global biogeochemical cycles. Glob. Biogeochem. Cycles 28, 71–85. doi: 10.1002/2013GB004705
Pörtner, H. O., and Farrell, A. P. (2008). Physiology and climate change. Science 322, 690–692. doi: 10.1126/science.1163156
Proctor, M. C. F., and Tuba, Z. (2002). Poikilohydry and homoihydry: Antithesis or spectrum of possibilities? New Phytol. 156, 327–349. doi: 10.1046/j.1469-8137.2002.00526.x
Scheidegger, C., and Werth, S. (2009). Conservation strategies for lichens: Insights from population biology. Fungal Biol. Rev. 23, 55–66. doi: 10.1016/j.fbr.2009.10.003
Smith, D. C., and Molesworth, S. (1973). Lichen Physiology Xiii. effects of rewetting dry lichens. New Phytol. 72, 525–533. doi: 10.1111/j.1469-8137.1973.tb04403.x
Tuel, A., and Eltahir, E. A. B. (2020). Why is the mediterranean a climate change hot spot? J. Clim. 33, 5829–5843. doi: 10.1175/JCLI-D-19-0910.1
Vallese, C., Nascimbene, J., Giordani, P., Benesperi, R., and Casazza, G. (2021). Modelling range dynamics of terricolous lichens of the genus Peltigera in the Alps under a climate change scenario. Fungal Ecol. 49:101014. doi: 10.1016/j.funeco.2020.101014
Keywords: lichens, LiBry, mechanistic models, growth, Lobaria
Citation: Di Nuzzo L, Canali G, Giordani P, Nascimbene J, Benesperi R, Papini A, Bianchi E and Porada P (2022) Life-stage dependent response of the epiphytic lichen Lobaria pulmonaria to climate. Front. For. Glob. Change 5:903607. doi: 10.3389/ffgc.2022.903607
Received: 24 March 2022; Accepted: 06 October 2022;
Published: 26 October 2022.
Edited by:
Knut Asbjørn Solhaug, Norwegian University of Life Sciences, NorwayReviewed by:
Yngvar Gauslaa, Norwegian University of Life Sciences, NorwayVera Androsova, Petrozavodsk State University, Russia
Copyright © 2022 Di Nuzzo, Canali, Giordani, Nascimbene, Benesperi, Papini, Bianchi and Porada. This is an open-access article distributed under the terms of the Creative Commons Attribution License (CC BY). The use, distribution or reproduction in other forums is permitted, provided the original author(s) and the copyright owner(s) are credited and that the original publication in this journal is cited, in accordance with accepted academic practice. No use, distribution or reproduction is permitted which does not comply with these terms.
*Correspondence: Paolo Giordani, giordani@difar.unige.it
†These authors have contributed equally to this work